An 11-Atom Sensor Sheds Light on the Quantum World
by Alan S. Brown
Sander Otte of Kavli Institute of Nanoscience Delft explores the edges of quantum mechanics
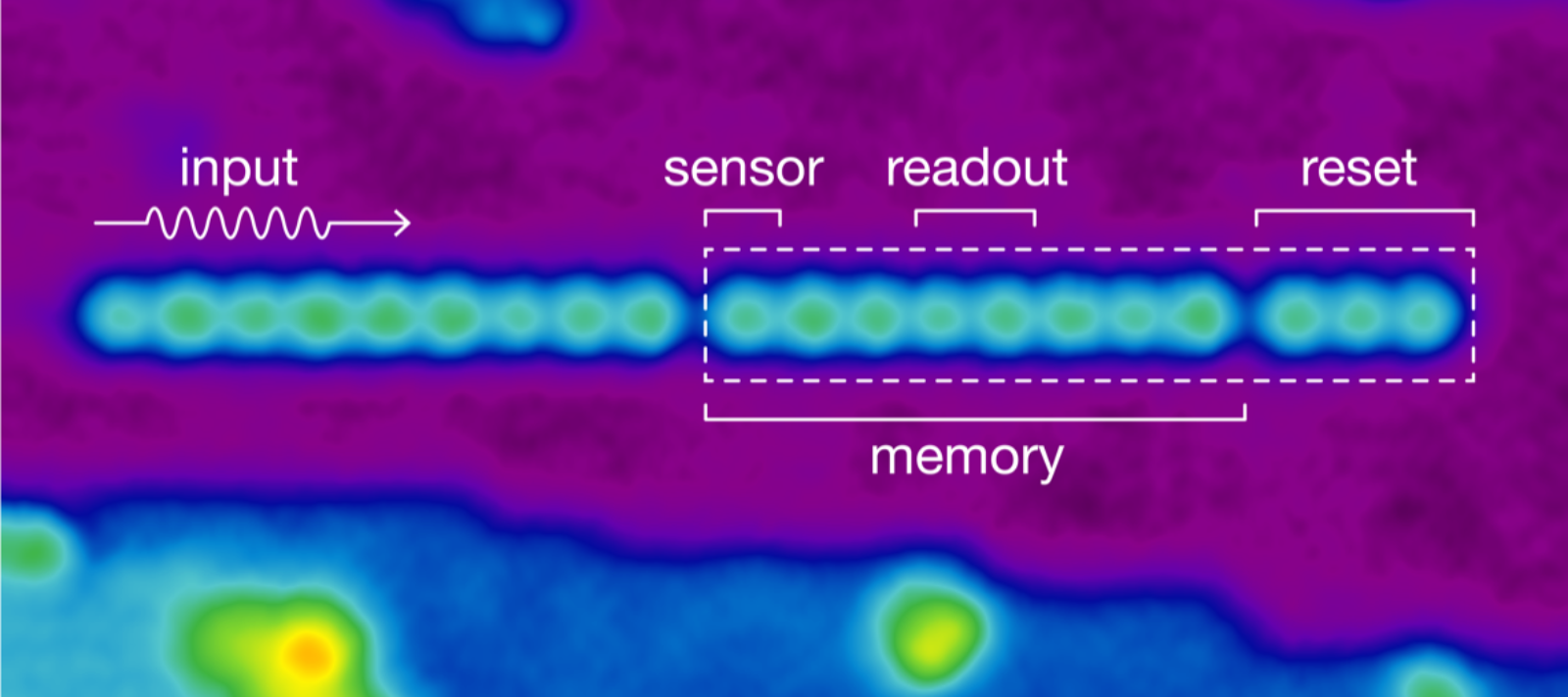
The Author
The Researcher
The world is awash in sensors so small, they tuck neatly into the slimmest smartphone. Yet these silicon slivers look gargantuan when compared with an 11-atom sensor developed Sander Otte, a member of the Kavli Institute of Nanoscience at Delft University of Technology in The Netherlands.
Although the sensor consists of only a few atoms, it contains all the functions found in commercial equivalents. This includes a sensing element, memory, data readout, and a “reset button” that enables it to make another measurement.
Otte built the sensor atom by atom to measure the rapid pulse of a single magnon, a quantum particle of magnetic energy, as it races down a short wire only one atom thick.
He fabricated the sensor element from eight iron atoms by coupling together their magnetic spins, a quantum property of subatomic particles that determine an atom’s magnetic moment (its strength and orientation). Once the spins were coupled and the moments aligned, the eight atoms performed as a single unit.
“We wanted to show that we could actually build a device out of individual atoms that was engineered so that the spins collectively do something useful,” Otte explained.
The device’s size is close to the point where materials make the tenuous transition from quantum behavior to classical properties. Physicists know little about this murky region, and that fascinates Otte.
We wanted to show that we could actually build a device out of individual atoms that was engineered so that the spins collectively do something useful.
Sander Otte, member of the Kavli Institute of Nanoscience at Delft
Manipulating atoms
It was 30 years ago that IBM physicists developed a way to use the electrical field generated by a scanning tunneling microscope (STM) to shovel individual atoms from one location to another. Otte remembers seeing an image of xenon atoms arranged to spell “IBM” and thinking, “That’s what I want to do.”
By the early 2000s, as a graduate student, he was doing just that. Otte, however, focused on atoms with intrinsic magnetic properties, such as cobalt and iron. While researchers could push around these atoms with the single atom at the end of the STM’s tip, they had no way to measure their magnetic properties.
Otte modified an STM to do just that. This enabled him to measure spin. After he received his Ph.D., Otte spent the next 15 years learning how to manipulate those spins.
“We learned that if you put an atom in a certain environment, it has a certain magnetic strength and excitation energy and a preferential orientation,” he said. “If you park one atom next to it, the coupling strength changes depending on the distance.
“Slowly, we expanded our toolbox of spin systems with coupled magnetic fields. We would put two atoms together, then add a third atom. We would start with iron atoms and add manganese atoms. Slowly, we learned how to create atomic structures that behaved the way we wanted it to behave.”
Eventually, he was ready to use that toolbox to build something.
Sensor
He soon found a problem that an atom-sized device could solve. When a magnetic material is excited, its magnetic moment starts to fluctuate. This fluctuation moves like a wave through the material and eventually releases quantum particles called magnons, units of magnetic energy similar to a photon, which are a unit of light.
Magnons move at tremendous speeds but dissipate quickly. The only way to measure them directly is with something small enough to interact with a passing magnon. That called for an atomic-scale sensor.
Conceptually, Otte’s device was simple. Otte excites a magnetic material with the tip of his STM. This generates a magnon that races down a “wire” nine atoms long to the sensor, which snaps like a mousetrap when the magnon passes through it. When he moves the STP tip over it, he can read whether it has tripped or not.
The device had four parts. The first the eight-atom sensor. It consists of a row of iron atoms whose spins, which can be either up or down, were coupled in an up-down-up-down-up-down-up-down configuration. When the magnon hits the first atom, it flips its spin to the down position. This change then flips the spin of the next atom to the “up” position. That changes the next atom in the line and so on until all eight iron atoms are flipped.
The eight iron atoms act like a memory. They hold their new spin positions for a few seconds, enough time for the STM to move over it and take a readout of the results.
Finally, the device had three additional atoms that serve as a reset button. They are located on the side of the sensor furthest away from the magnon source. When Otte energizes the reset with the STM, he generates a magnetic field that flips the sensor spins back to their original position.
The eight atoms that make up the sensor element are just on the edge of where the quantum world meets classical physics. They are small enough to respond to a quantum magnon particle by flipping, but large enough to remain stable for the few seconds it takes to move the STM tip over them and take a reading.
Yet the quantum world keeps poking that sensor. Although Otte runs his experiment at close to absolute zero to minimize random energy, every once in a while, a random quantum jump will flip his sensor anyway.
“If we were to add two more atoms, so you get 10, its stability would increase by orders of magnitude,” he said. “Yet, at four atoms, we’re deep into the quantum regime. At 12 atoms, we’re deep in the classical regime. So this is something in between.”
Otte hopes to learn more. Spins, he says, are the simplest quantum system.
“If we can control them, we have the most elementary quantum building block at our disposal, and then you can build anything,” he said. “That’s why I’m so fascinated by spins, because it’s a prototype for almost any other sort of quantum mechanics.”
His sensor, which sits on the edge of that world, is just a start for probing it.