2014 Kavli Prize in Astrophysics: A Discussion with Alan Guth, Andrei Linde and Alexei Starobinsky
by Kelen Tuttle
The winners of the 2014 Kavli Prize in Astrophysics discuss their development of the theory of inflation and reflect on how it has changed our view of the universe.
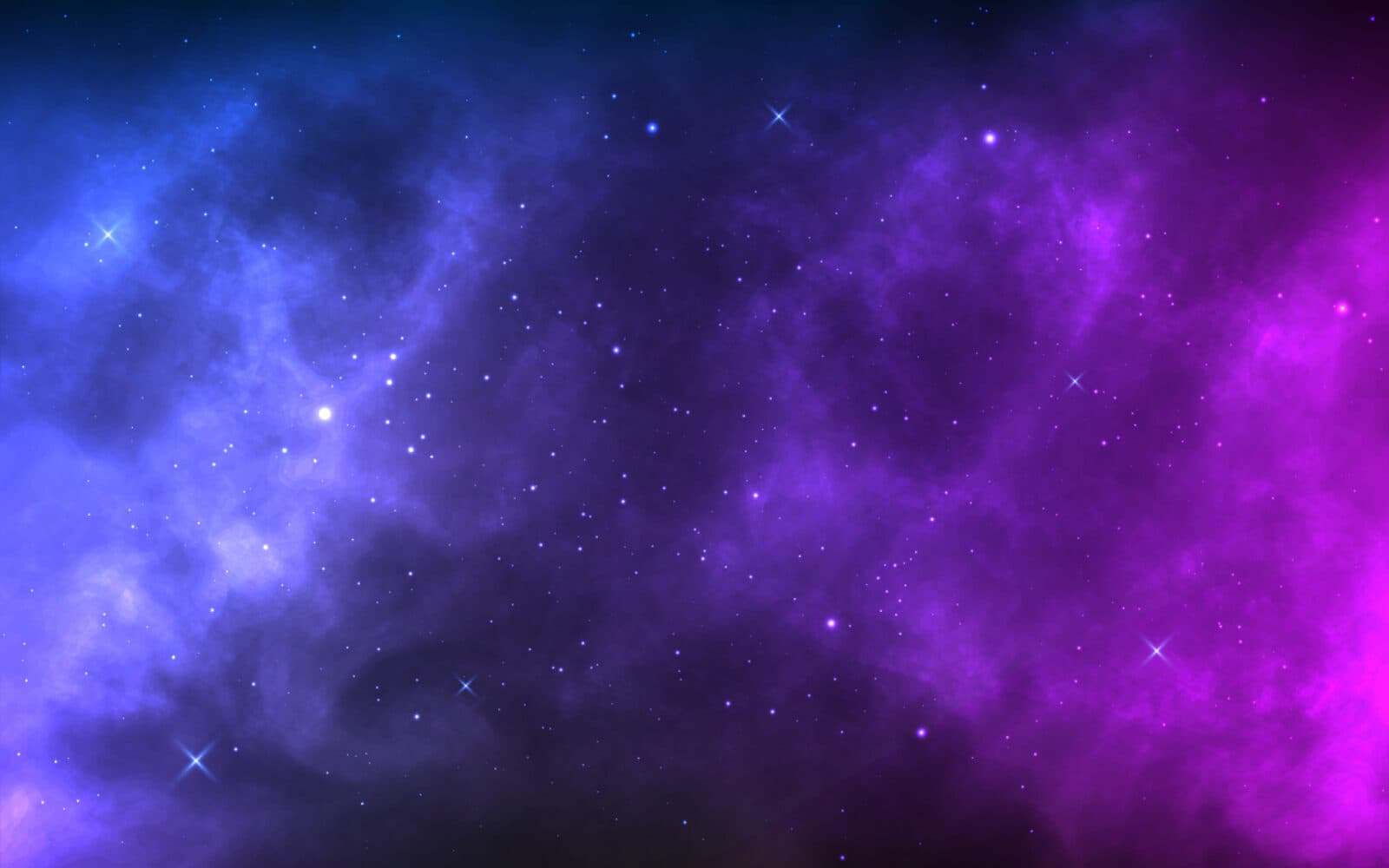
The Author
The Researchers
Over the course of several years, the 2014 Kavli Prize Laureates in Astrophysics –Alan Guth, Andrei Linde and Alexei Starobinsky – led the development of a strikingly simple and elegant explanation. They postulated that, almost 14 billion years ago, our infant universe exploded in a sudden and violent burst, doubling in size more than 60 times in less than a billionth of a trillionth of a trillionth of a second. The sudden expansion explains why the universe is so homogeneous over great distances, and how very faint inhomogeneities created by quantum fluctuations during the sudden expansion can become the seeds from which galaxies grew.
For pioneering the theory of cosmic inflation, Drs. Guth, Linde and Starobinsky will receive the 2014 Kavli Prize in Astrophysics.
- ALAN H. GUTH – Victor F. Weisskopf Professor of Physics and MacVicar Faculty Fellow at the Massachusetts Institute of Technology.
- ANDREI D. LINDE – Harald Trap Friis Professor of Physics at Stanford University.
- ALEXEI A. STAROBINSKY – Principal Research Scientist at the Landau Institute for Theoretical Physics in Moscow.
Ahead of the 2014 Kavli Prize Ceremony, the 2014 Kavli Prize Laureates in Astrophysics held a conversation with science writer Kelen Tuttle and talked about the process of developing the theory of inflation and its implications on our understanding of the universe as a whole. The conversation has been amended and edited by the laureates.
THE KAVLI FOUNDATION: Alan, Andrei and Alexei, what do you think has been the biggest impact of the theory of cosmic inflation? How significantly has it changed our view of the universe?.
ALAN GUTH: I would say that inflation really casts cosmology in a totally different light. With inflation, we suddenly have a tool for being able to predict what the physics in the early universe looked like. That process is by no means complete – we really need a full theory of physics at arbitrarily high energies before we'll know exactly what inflation predicts. But what we have found is that the simplest versions of inflation – that is, based on the simplest assumptions about the way that physics might behave at very high energies – make real predictions for the appearance of our universe, predictions that actually work to a very high degree of accuracy. And I find the whole thing kind of astounding. I'm just amazed at how good the agreement is.
ALEXEI STAROBINSKY: From my point of view, I would say what we have discovered is something that's as big as the Big Bang theory. More specifically, we found a new epoch in the history of our universe that came before the hot Big Bang. It's one more step in the understanding of our universe, one more step in discovering its remote past.
It's also very important because with this theory, cosmology becomes more like other areas of physics in that it becomes predictive. With the initial conditions predicted by inflation, we can extrapolate the whole subsequent history of the universe. In particular, we can predict what we see today with great accuracy. That's very exciting. And it also makes cosmology as standard – from the point of scientific methodology – as particle physics or condensed matter physics.
ANDREI LINDE: From my perspective, what happened was actually a tremendous change in perspective, both in the macro and the micro scales. Before the theory of inflation, everyone thought that quantum mechanics had an effect at very small scales, but at large scales it was considered not to be relevant. But we learned that the largest objects in the universe – galaxies – were produced by what’s called quantum fluctuations. These random changes associated with the general unpredictability of quantum theory occurred at the very early stages of inflation. Some time ago, this would have sounded like a crazy idea, one that's good for science fiction books but not for physics. And that would explain why for many years, everyone looked at the theory of inflation with this feeling of awe and caution simultaneously. Yet you go and measure and everything just fits into this science fiction picture. It's just amazing.
TKF: According to the theory of cosmic inflation, the entire universe was almost unimaginably small in its first moments. Why is this important?
ALEXEI STAROBINSKY: It is simpler to say that the part of the universe that we see now was unimaginably small, less than about 0.0000000000000000000000000001 – that’s 27 zeros between the decimal point and the 1 – centimeters across, if we take at face value the most recent astronomical data on the heat left over from the Big Bang known as the cosmic microwave background. The question about the size of the universe as a whole requires a separate explanation and is not as closely related to observations.
At this small size, all of the universe that we can see today was causally connected. It is this property that gives us a possibility to use causal laws and the principles of physics to determine the initial conditions of the universe.
TKF: And Andrei, what does inflation theory say happened in the early universe – the period when the universe as we know it was just an infant?
ANDREI LINDE: In the simplest models of inflation, the size of the observable part of the universe at the beginning of inflation should have been at least 14 orders of magnitude smaller than the proton size – absolutely minuscule. Inflation takes this tiny speck of space, expands it and produces everything that we see right now. It’s really quite amazing.
TKF: Alan, the rapid expansion described by inflation solves some problems of the standard Big Bang theory – in particular, the fact that there is a uniform structure to the universe. Can you explain these problems?
ALAN GUTH: There are three main problems with the conventional Big Bang theory that inflation solves. One of them is usually called the flatness problem, and it concerns the expansion rate of the early universe. If we consider the expansion rate, at for example one second after the beginning, its value would have to have been exactly right to an accuracy of 15 decimal places. If the expansion rate had been just a tiny bit smaller – by changing the 15th decimal place to be just one less than we think it was – then the universe would have recollapsed before any structure could have formed. If the expansion rate had been just a little larger – by an increase of one in the 15th decimal – then the universe would have flown apart so quickly that no structure could have formed. So, for the universe to look anything like the way it does, the expansion rate one second after the beginning had to be just right, within this incredibly narrow range. But without inflation, we don't know any reason why it should have been in this range. We call this the flatness problem because general relativity implies a connection between the expansion rate, the density of matter, and the curvature of space. The “just-right” value of the expansion rate is exactly the value that corresponds to the absence of curvature, and hence a “flat” universe.
Inflation solves this problem because inflation includes a description of the force that drove the expansion of the Big Bang. And it turns out that this force drives the universe to exactly the required expansion rate.
The second problem is often called the horizon problem. It's the problem of understanding how the universe got to be so uniform. The uniformity of the universe is seen most clearly in the cosmic microwave background radiation – the afterglow of the heat of the Big Bang that Alexei mentioned earlier. This radiation has by now been measured very precisely, and its intensity is uniform in all directions to an accuracy of one thousandth of one percent. But how did it get this way? Without inflation, it is hard to see how it could have happened. Suppose we consider two of the photons that make up this radiation, coming from opposite directions in the sky. We can trace these photons back to when they were emitted, about 380,000 years after the beginning. When we do that, we find that the two sites of emission were separated from each other, at the time of emission, by about a hundred times the distance that light could have travelled up until that time. Since nothing, as far as we know, can travel faster than light, there was no way that either of these sites could have received any information from the other. But without communication, what could possibly cause them to look so similar? That was a big mystery in the context of conventional cosmology. Inflation solves this problem by inserting into the history of the universe a period of exponential expansion. Before that growth spurt, the presently observable part of the universe was vastly smaller, and so during this period before inflation there was plenty of time for the uniformity to become established. Inflation then stretches this tiny region of uniformity to become large enough so that today it encompasses everything that we see.
The third problem is the non-uniformity that we do see. As I mentioned before, the intensity of the cosmic microwave background is uniform to an accuracy of one thousandth of one percent. But the intensity has now been measured to much better accuracy, and it is found that it varies from one direction to another, within a range of about one thousandth of one percent above and below the average. And these differences are critically important to the evolution of the universe because they lay the seeds that ultimately give us all of the structure that we see in the universe. According to inflationary models, these variations were generated by quantum fluctuations during the inflationary period, causing inflation to end at slightly different times in different places. Because of these quantum effects, the mass density of the universe is slightly higher in some places and slightly lower in others – causing the entire structure of the universe that we see around us today.
"...[W]hat we have discovered is something that's as big as the Big Bang theory. It's one more step in the understanding of our universe, one more step in discovering its remote past." – Alexei A. Starobinsky
TKF: Let's talk about how you all got started on this theory. Alexei, when you originally came up with the idea of cosmic inflation, you were focused on problems other than the ones that Alan just described, is that correct?
ALEXEI STAROBINSKY: In the 1970s, I was trying to figure out what may have occurred at the beginning of the hot Big Bang. To that end, I was investigating the effects of the creation of elementary particles and antiparticles that occur due to the fact that, according to Einstein, space-time is curved.
However, the general opinion at that time was that all such effects would be exceedingly small, if they exist at all, so there was no hope that any experiment or observations would ever be able to check these academic studies. The situation changed completely in 1979 when, considering the creation of gravitons – elementary particles associated with the gravitational field – as an example, I discovered that if the period of exponential expansion of the universe for which Alan later gave the famous name “inflation” preceded the hot Big Bang, then these gravitons should exist at all scales, including astronomical and cosmological ones, in the form of either particles or fluctuations of the space-time metric of the universe. Moreover, it should be quite possible to measure these fluctuations and, in this way, to confirm or disprove the idea of inflation.
So, in this way, not only does inflation offer an explanation of enigmatic things previously known, but it also challenges astronomers with the ambitious task of engineering great observational projects that aim to discover and measure the predicted fundamental effects – and to get prizes for these discoveries. And this has actually occurred over the past 20 years, and more and more predictions of the inflationary theory have been confirmed.

This ability to test the theory was the second reason why I became interested in it. The first reason was the theory’s aesthetic beauty: the universe during inflation was as maximally symmetric as the fundamental laws of physics permit! To obtain all results of my 1979 paper, I didn’t need any model of inflation; the simple hypothesis of its existence in the past was sufficient. However, this was not enough for the quantitatively correct calculation of formation of structure and all compact objects in the universe: galaxies, stars, planets, and even ourselves – this can be done using some concrete model of inflation only. That is why after that I began to think about some internally self-consistent theoretical model in which the universe not only went through the inflationary stage, but it could also safely leave it without destroying advantages of inflation and enter the previously known standard hot Big Bang epoch. I indeed found such a model, which I published in 1980. Using this model, the following year Mukhanov and Chibisov made the first quantitative calculation of formation of structure in the universe. One prediction of this process was recently confirmed by the WMAP and Planck experiments. So this model still remains viable after 34 years, apart from the most recent result of the BICEP2 experiment, which still has to be confirmed.
TKF: Alan, what about you? I understand that you were a bit of an outsider to this area when you were first introduced to some of the problems you described a moment ago.
ALAN GUTH: I got into it entirely through the influence of a friend of mine, Henry Tye, who was at the time a fellow postdoctoral physicist and is now a physics professor at Cornell University, and the Director of the Institute for Advanced Study at the Hong Kong University of Science and Technology. He was interested in magnetic monopoles, which are hypothetical elementary particles that have only one magnetic pole, and how many of them could have been produced in the Big Bang. As a result of these investigations, we came up with the idea that perhaps the early universe underwent an extreme supercooling – that is, perhaps the universe cooled to temperatures far below the transition temperature of a theoretically predicted phase transition, without the phase transition taking place. As an analogy, water can be supercooled to temperatures as low as 40 kelvin below its normal freezing temperature, without it turning to ice.
I went home one night, I'm pretty sure at Henry's suggestion, and considered the ancillary question of whether or not this supercooling would affect the expansion rate of the universe. Once I wrote down a few equations – it really was in fact pretty simple – it became obvious that those equations were saying that such a large amount of supercooling would cause the universe to expand exponentially.
I realized the same night that this exponential expansion would solve the flatness problem. So I immediately became incredibly excited about it, and the excitement rose a little bit a few weeks later when at lunch in the SLAC cafeteria people were talking about this thing called the horizon problem, which I had never heard of. It was immediately clear that this process of exponential expansion would solve that problem too. So I became all the more excited. After that, I started giving talks about it and eventually wrote it up, and it paved the way toward what we now call inflation..
TKF: And from there, Andrei, you picked up the theory and proposed a series of ever more sophisticated working models. What did those modifications add to the theory?
ALAN GUTH: Actually, Andrei might be too modest to say it, but what you said is an understatement. Andrei didn't invent more working models. He actually invented the first working model. My version of inflation did not work. Andrei made it work.
"When people said that gravitational waves would be the smoking gun for inflation, my response was that I thought the room was pretty filled with smoke already." – Alan H. Guth
ANDREI LINDE: I came to this field from particle physics and then I got interested in the early universe. At the time, I did not know about the horizon and flatness problems that Alan just described, nor did I know anything about Alan's work. I knew about the work by Alexei Starobinsky – it was quite famous in Russia – but the question that was addressed by the work by Alexei was different from solving the problems of flatness and horizon.
Then, shortly after I heard about the flatness problem for the first time, someone called and asked me if I had received the paper by Alan Guth, and seen how he solved it. I told him no, I didn't receive it, but let me explain to you what it's about and why it does not work. And so it was kind of funny, because I knew what was going to be there, but I did not have the paper yet.
What Alan was able to do, he was able to bring together these problems of cosmology and knowledge about the conditions of the early universe, making it into a scenario that inspired many people.
For quite a while I was trying to think about how to improve his scenario and that was very challenging – so challenging, in fact, that I think this is why at this time I had an ulcer. When you have a totally disturbing problem and an absolutely stunningly beautiful idea of how to solve it, but you cannot make it work – that’s just painful.
Eventually, though, I found a way to resolve some of the problems, to make things happen in such a way that we could predict what we see today. This new inflationary scenario existed for about a year, until we learned, due to some work by Alexei, Alan and several other people, that this scenario is also imperfect. The quantum fluctuations produced in this scenario were too large, so we needed to replace it somehow.

In 1983, I proposed another scenario, which was called “chaotic inflation.” It deviated from the previous ones pretty substantially, making everything much simpler from the conceptual point of view while keeping the main idea of having something like exponential expansion in the early universe. In this scenario, we do not need any assumptions about the cosmological phase transitions, supercooling, or even the very idea that the early universe was hot after the Big Bang – and yet this scenario works.
Most of the versions of inflationary theory that we have now are based on ideas similar to the scenario from 1983. Since that time, inflationary theory has become more understandable, more streamlined, simpler – so much so that now, looking back, it’s a surprise that nobody was thinking about it earlier. But it’s only simple looking back in the rearview mirror.
TKF: Was there any collaboration among the three of you as the theory progressed, or did each of you work independently, seeing one another's work only after it was published?
ANDREI LINDE: There wasn't very much formal collaboration. I collaborated with Alan only once, and that was just a few years ago. I collaborated with Alexei several times, but that was after inflation was invented. When we developed the theory, mostly we were working separately.
ALAN GUTH: But we were communicating some. We certainly met at workshops and talked.
ALEXEI STAROBINSKY: Indeed. We had very different approaches, and we were coming from different backgrounds: particle physics for Alan and Andrei, and astrophysics and cosmology for me. This was important and complementary. Regarding collaboration, as Andrei said, we produced a number of top-cited papers jointly with our very talented colleague Lev Kofman, my former and probably the best student, who is, unfortunately, not with us anymore.
ANDREI LINDE: I still have one unfinished paper with Alexei that I carry with me on my computer even now. Hopefully we will return to it eventually!
TKF: Another collaboration, BICEP2, earlier this year announced that it had detected distinctive twisting patterns in the earliest light we can detect. These patterns match what would be expected if gravitational waves – which, Alexei, you were the first to postulate – interacted with matter and energy in the early universe. Yet it's since become unclear if what they're seeing really is a signal from the early universe or if perhaps instead they're seeing cosmic dust that's mimicking the expected signal. Everyone is now waiting for follow-up measurements that will either confirm or deny the result. What have the past few months been like for each of you? I imagine it's been a bit of a rollercoaster.
ANDREI LINDE: I have to say that the way this story was portrayed in the media was kind of out of control. It was presented as if the discovery of gravitational waves would be the smoking gun for inflation. And this is a very smart way of formulating the story but it’s also dangerous and misleading. Some reporters, trying to emphasize the significance of the BICEP2 results, even said that previously inflation was just an interesting but unproven theory without any observational support, and now it was finally confirmed. It is true that the discovery of gravitational waves would give an additional very strong and important confirmation of inflation. But we already have lots of observational evidence in favor of inflation, and not discovering gravitational waves would not make the theory of inflation wrong. And so the way these results were presented to the general public has been somewhat misleading.
ALAN GUTH: I agree with everything you say. When people said that gravitational waves would be the smoking gun for inflation, my response was that I thought the room was pretty filled with smoke already. There's a lot of evidence for inflation that's been dismissed in the recent general news articles, including predictions that it makes about the mass density of the universe, predictions about the spectrum of the fluctuations that are seen in the cosmic background radiation – that is, measurements of how the intensity of the ripples in the background radiation varies with the angular size of the ripples as seen on the sky – and predictions about the statistics of these fluctuations, called gaussianity. All three of these are more complicated and harder to explain in the general press – but they are just as important as the gravitational waves.
"Inflation explains why our universe is so big, why it's so uniform, why it's not rotating, why it's similar in all directions." – Andrei D. Linde
ANDREI LINDE: I would add that these are just three of the predictions that have been observationally confirmed. There are many other, more technical ones as well. And beyond the specific predictions, this scenario has such important and strong explanatory power. Inflation explains why our universe is so big, why it's so uniform, why it's not rotating, why it's similar in all directions. And as of now, we do not have any well-developed explanations that compete with inflation, despite many attempts.
ALEXEI STAROBINSKY: I am looking forward to independent measurements to compare to the BICEP2 result. Like the discovery of the Higgs boson, which was made by two independent collaborations, this discovery requires independent verification. That's especially true because BICEP2 made measurements only at one frequency; we need to test the signal at other frequencies as well to prove its relation to gravitational waves from inflation. And that test is coming, from the Planck satellite and others.
Like many others, I think it’s likely that the BICEP2 result is partially contaminated by nearby sources, mainly by the emission of galactic dust, but it is not clear at present to what extent. Either way, primordial gravitational waves generated during inflation should exist. The question is only when their discovery will be confirmed by independent observations and what will be the right value of quantity “r,” which describes the strength of these gravitational waves. Inflation predicts that r should be small, but we are prepared for its value to be both around 0.1 – as in one of the models proposed by Andrei – and 25 times less – as in my 1980 model. Just as the measurement of the Higgs mass – the value of which was not, by the way, predicted by the Standard Model of elementary particles – helped to say many new things about this model and its possible extensions, the final measurement of r will provide us with much new and remarkable information about details of inflation.
ANDREI LINDE: I should say that no one is questioning the BICEP2 results – these are absolutely record-breaking. It’s the interpretation of the data that’s now in question. Is it due to gravitational waves, or due to dust? We look forward to independent verification.
Also, as Alexei says, it’s important to realize that inflationary theory generally predicts these gravitational waves, but the strength of the waves is model-dependent. Each model predicts a specific value. So while the discovery of gravitational waves would indeed be the smoking gun for inflation, non-discovery of gravitational waves, at this point, would not mean much. It would rule out some specific versions of the inflationary theory, but most versions would remain viable.
Following Alexei, I would bring up the recent progress of particle physics as an analogy. Half a century ago, a new principle of construction of consistent models of weak and electromagnetic interactions was discovered. It required the existence of the Higgs field. Without this field, the theory was inconsistent, suffering from many different problems. This was a major revolution in the theory of elementary particles. But there are many different versions of this theory, and dozens of free parameters to be determined experimentally.
Similarly, inflation is not just a single model. It is a new cosmological paradigm, a set of ideas which allow us to construct consistent cosmological theories. And then we use observations to find which of these theories best describes the world where we live.