A Boiling Hot Cosmic Stew
by Adam Hadhazy
Research Highlights from Kavli Astrophysics Institutes
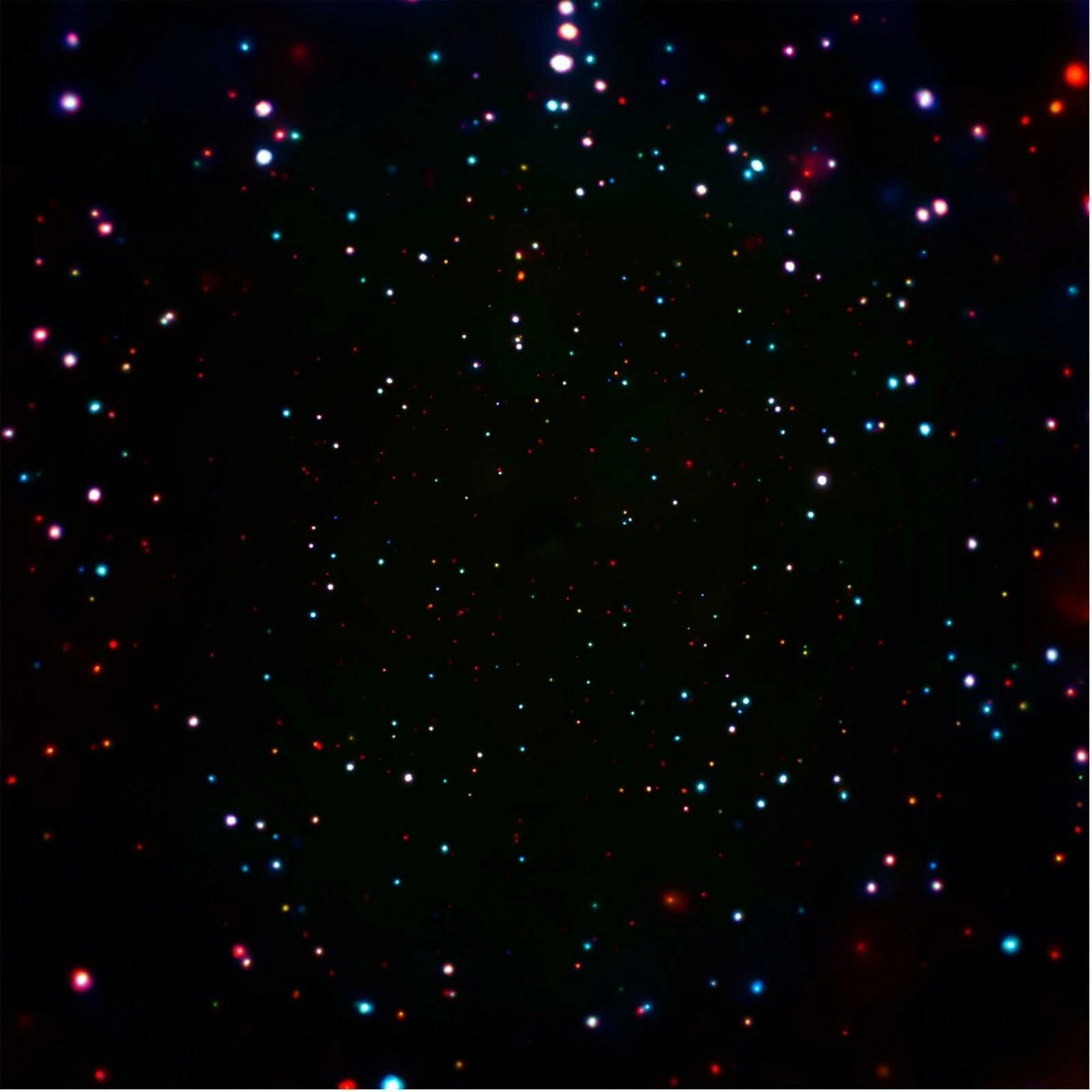
The Author
At Kavli Astrophysics Institutes, researchers push the boundaries of our knowledge and capabilities of science at the largest of scales.
The first "bubble" of a boiling hot cosmic stew
Although we appropriately think of space as a cold place—and indeed, most of it barely registers above absolute zero—there is a gargantuan amount of material in the sprawling expanses between galaxies that is instead hot. Quite hot, that is. This so-called warm–hot intergalactic medium (WHIM) contains about 90 percent of all atoms in the universe, in fact, and in the form of ionized hydrogen gas. The temp of this WHIM ranges from around 100,000 degrees Celsius to greater than 10 million degrees Celsius. By way of analogy, the WHIM can be construed as a hot stew within which galaxies are "chunks" (keep in mind that the stew bowl contains billions upon billions of such far-flung chunks). Researchers at the Kavli Institute for the Physics and Mathematics of the Universe (Kavli IPMU) at Tokyo University have now found what can be thought of as one of the very first "bubbles" in this boiling cauldron. The researchers found what seemed like a run-of-the-mill galaxy protocluster from when the universe was only 3 billion years old that. When examined further by the Keck-I Telescope in Hawaii, the protocluster turned out to not have an absorption shadow characteristic of cold gas. Rather, the galaxy conglomeration has an ambient gas temperature of about a million Celsius, and thus more in line with the modern universe. The discovery will help advance our understanding of cosmic evolution.
Steps forward for cutting-edge x-ray sensors
X-ray telescopes are very well-suited to studying black holes, which emit copious high-energy x-rays outside of their event horizons (the boundary within which light cannot escape the object's gravitational clutches). To enhance this capability, a team of scientists is developing powerful new sensors. The team consists of researchers at the Massachusetts Institute of Technology's (MIT) Kavli Institute for Astrophysics and Space Research (MKI) and the Kavli Institute for Particle Astrophysics and Cosmology (KIPAC) at Stanford University. The scientists have their work cut out for them, seeing as these sensors must keep up with the expected 10-30 times greater x-ray-collecting abilities of next-generation x-ray space telescopes. Furthermore, the new sensors must be ultra-sensitive to detect lower-energy kinds of x-rays. Toward this end, the sensors must have extremely little internal "noise". And as always with gear intended for operation in space, the sensors are severely constrained on size, mass, and power consumption. Progress is thankfully good, involving new sensor structures, manufacturing techniques, and low-power electronics. The future indeed looks bright for x-ray astronomy.
Could black holes be "observing" the rest of the universe?
A story by Quanta explores the fascinating perspective of a new paper coauthored by Robert Wald, a senior member of the Kavli Institute for Cosmological Physics (KICP) at the University of Chicago. The paper explores how black holes can in effect "observe" the universe in a way that causes decoherence—the concept in quantum mechanics whereby any given particle goes from existing in a state of possibility to existing as a measured definitiveness. Black holes theoretically can cause this decoherence because of their event horizons. Supposing a given particle is in superposition—the state of possibility—outside of the black hole, and a quantum of information from the particle, such as gravitational or electromagnetic radiation, enters the black hole. Even at great distance from the black hole, the particle would decohere by virtue of the black hole "observing" a property of the particle via that received quantum. The ramifications of the paper's thought experiment remain up for debate. But the researchers hope that further investigating the phenomenon could lead to insights connecting quantum physics and general relativity—two supremely well-supported descriptions of the physical universe at different scale that famously and unfortunately, are incompatible.
On the origins of an astrophysicist
A profile story relates how George Ricker, a senior research scientist at MKI, went from building his first telescope in third grade in rural Florida to spearheading NASA’s Transiting Exoplanet Sky Survey (TESS), a planet-hunting spacecraft that has plied the skies since 2018. Originally at MKI, Ricker worked on and founded a lab dedicated to special light-sensing detectors based on silicon charge-coupled devices (CCDs). The sensors helped move x-ray and gamma-ray astronomy forward, among other advances. Ricker and his group then formulated the idea for TESS in 2006. The telescope scans about a quarter-million nearby stars for faint dimming caused by the passing of hosted planets across their stellar faces. In total, pulling off the TESS mission took more than 6 million person-years, Ricker says in the story. The spacecraft has found about 6,000 candidate exoplanets and is identifying some of the closest alien worlds as the best candidates for detailed study by JWST and other observatories.
Building up Stars 2.0
Compositionally, the very first stars had to work with a very limited elemental menu—almost entirely hydrogen, a bit of helium, and a trace of lithium, all formed in the Big Bang. Though the process of nuclear fusion that makes stars shine and then through explosive end-of-stellar-life supernovae, this first generation of stars produced a bunch of heavier elements that went on to expand the menu for the second generation of stars. The second generation of stars then proceeded to produce even more heavier elements for the third generation of stars, like our Sun, supplying enough heavier elemental material to support the complex chemistry of life. Researchers have long sought to nail down the expansion of the elemental menu from the first to second generations of stars, and a new study has advanced that goal. Researchers at Kavli IPMU and colleagues studied a batch of more than 450 second-generation stars right here in our Milky Way. Compared to later stellar generations, these particular stars are considered extremely "metal-poor"—astronomer-speak for being deficient in elemental content beyond helium. The researchers employed a kind of artificial intelligence, called machine learning, which surmised that over two-thirds of the stars had been chemically enriched by multiple previous supernovae. The findings help fill in the history of these stars, indicating they evolved in close quarters in star clusters with other stars that exploded and enriched their neighbors.