A Camera Fast Enough to Stop Light in Its Tracks
by Alan S. Brown
Lihong Wang developed a camera so fast, it can freeze the path of light or image biological events as they happen
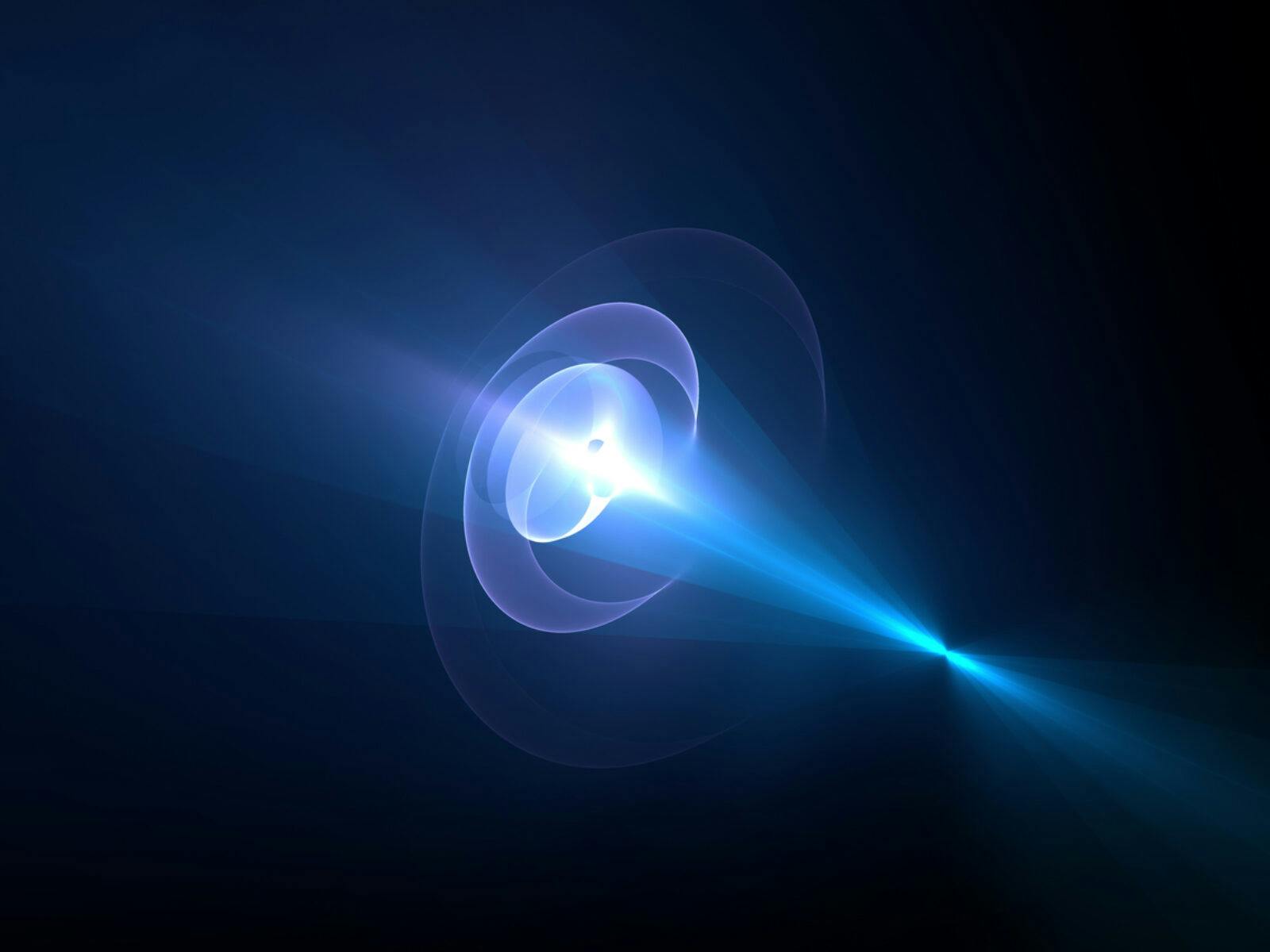
The Author
The Researcher
When something happens quickly, we say it takes place in the blink of an eye. Yet, during the 0.1 seconds it takes to blink, a camera developed by Lihong Wang can take 7 trillion pictures.
This is fast enough to stop the motion of burst of light as it ricochets off mirrored walls or freeze proteins in their tracks as they change their shape as they react with other molecules. Fast enough to test hypotheses that were impossible to verify before.
Wang is a member of the Kavli Nanoscience Institute at Caltech, where he is a professor of medical and electrical engineering. He is a noted developer of optical systems, including photo acoustic computed tomography, which uses ultrasound to pinpoint tumors by reading the location of molecules that have been excited by lasers. The work, just reaching commercialization, could enable doctors to take breast mammograms without discomforting compression or find study tumors without exposure to radiation.
Wang’s new compressed ultrafast photography (CUP) camera could have even greater impact. Wang first introduced CUP in 2014 and has spent years upgrading its speed and capabilities. It has enabled his team to observe events that would have looked like blurs on other ultra-high-speed cameras and promises to change the way researchers view the world.
Seeing the light
Take, for example, Cherenkov radiation, the light created when a charged particle passes through matter faster than light moves through the same material. (Light moves at the speed of light only in a vacuum but slows down as much as 25 percent in other media.)
“Theory predicts that the charged particle creates a cone of light, the way an airplane breaking the sound barrier generates shock waves in the air,” Wang said. “But no one has ever observed what the cone looks like. We were able to use the CUP camera to create images of the exact structure of this light as it evolved.”
Understanding the structure of Cherenkov radiation would enable physicists to dive deeper into its theory and potential applications.
Three years later, in 2020, Wang turned his camera on optical chaos. Chaos, by its very definition, involves non-repeatable events. Once, this was a hot academic topic with potential implications for physics, communications, and cryptography. Interest petered out because experimentalists had no way to validate any hypotheses.
CUP could be the tool they needed. To demonstrate, Wang’s team created a polished cavity with a half-mushroom profile. Then he shot a very short pulse of laser light into the cavity. Using a CUP camera running at one billion frames per second, he was able to image the path of the light as it bounced off one wall and then another. This showed that the camera could give physicists a way to test their hypotheses.
Biological events may not move at the speed of light, but they take place blindingly fast. One example is the propagation of electrical pulses from one neuron to another in our brains. CUP can potentially image how, exactly, this process takes place. The data could be used to better understand how memory, cognition, and consciousness.
Whether the study involves fast-moving light or slower processes, CUP is a steep change from previous high-speed cameras. Those cameras are limited by the amount of information they can store in memory and the readout speed of their CMOS and CCD semiconductors.
At best, they achieve imaging speeds of millions of frames per second. This is fast enough to unlock many physical mysteries. Yet they cannot keep up with such extremely fast phenomena as ultrashort light propagation, radioactive decay, soliton formation, shock wave propagation, nuclear fusion, and biological events.
Compressed sensing
At first, researchers tried to get around speed limits by taking a snapshot of an event, then repeating the experiment and photographing it a short period of time later. They did this over and over again, stringing the photos together to create a movie of the event.
“If the event is highly repeatable, you can synthesize the movie and get some understanding of what’s going on,” Wang said. “But something like optical chaos by its very definition is not repeatable—and it’s happening at light speed. If you don’t have a fast enough camera, you just cannot study the event in a coherent way.”
The next big step forward was the streak camera, which measures the intensity of ultrafast light or radiation with time through a slit positioned alongside the event being filmed. First, the streak camera gathers light through a narrow slit. It then converts those photons into electrons and sends them through an electric field. This deflects the electrons onto a phosphor screen, where they form a two-dimensional map of the event. The horizontal (x) axis on the phosphor provides the photon’s position, while the vertical (y) axis shows the time it arrived.
Wang first learned about streak cameras after earning his doctorate at Rice University, where he studied under Frank Tittel and Nobel Prize winners Robert Curl and Richard Smalley (the discoverers of soccer ball-shaped carbon buckyballs). For his post-doctoral work, he decided to apply what he had learned about lasers to medical imaging.
“The streak camera was a technology that allowed you to image really fast, but only in one dimension because the vertical axis was reserved for time,” Wang said. “But I kept it in mind for more than a decade and finally decided to revisit it. We bought a streak camera and said, ‘There has to be something we can do to make it better.’“
Wang’s challenge was to find a way to open the slit and use the y-axis to provide spatial information without losing his temporal data along the way.
Wang’s solution involved a combination of encoding and data compression. Before injecting the image into the camera, Wang encoded the photons using a digital micromirror device. Its one million micron-scale mirrors created a pattern, or code, that enabled him to locate the position of each photon in two-dimensional space while taking advantage of the streak camera’s ability to segregate those photons based on the time they arrived in the camera.
Wang also used compressed sensing, which works because most images have what he calls “redundant information.”
“Imagine a white wall,” Wang said. “If I take a picture that includes that wall, I don’t have to record every white pixel, do I? I just need to record the boundary of the wall. I can save a lot of pixels by doing that.
“If I have a video, I can compress even harder because adjacent frames are highly correlated and not everything in those frames is moving so fast. Whatever we’re measuring is moving a little bit from frame to frame, but everything else is static, right?”
The result was a system that could get around some of the readout and memory limitations of semiconductor chips used for image processing—and process hundreds of billions of images per second.
New visions
Over time, Wang’s lab has added many new features to CUP, enabling it to capture trillions and then tens of trillions of images per second. His team has also created a variety of CUP flavors, enabling it to do polarization imaging and spectroscopy. This has made the technique valuable in a broader range of disciplines.
Although CUP remains expensive, Wang believes the concept could be implemented in low-cost lab devices and possibly even high-end consumer cameras.
Meanwhile, the race is on to push CUP speeds into the hundreds of trillions of images per second. In the 1000 terahertz range, CUP would be fast enough to see the oscillations of light.
Wang is not sure he can do that, but he foresees a big payoff if he can. “It’s like watching a horse race,” he explained. “We need to video it at 30 Hertz or more—30 frames per second—so we can see what’s going on.
“If we do it at 1 Hertz, we’re not going to be happy with the results. One second the horse will be here, the next moment it’s there. We’re seeing time averaged, instead of how the horses actually move. That’s what we want to do with light frequencies. Today, we’re seeing time averaged, not the actual phenomenon itself.”
Faster cameras may also help us understand the quantum world, and especially entanglement. Mathematics show that two entangled waves in distant locations can act as if they are one. That suggests some sort of communication between them. Einstein called it spooky action at a distance because it could not be explained. Perhaps ultra-high-speed images of those interactions might give us a deeper, more visceral understanding of such interactions, which can now be explained only by mathematics.
CUP may unveil other unexplored characteristics of light as well.
“I think our current understanding of light can potentially be advanced further,” Wang said. “I feel like we should never stop thinking, stop exploring until we truly understand. I’m a believer that there is always a deeper mystery to be uncovered.”
And at 70 trillion images per second, Wang is well on his way to uncovering it.