A Cradle for Enzymes Could Redefine Biodegradable Plastics
by Alan S. Brown
A new technology promises to break plastics down into molecules that feed soil bacteria and eliminate the challenge of microplastics
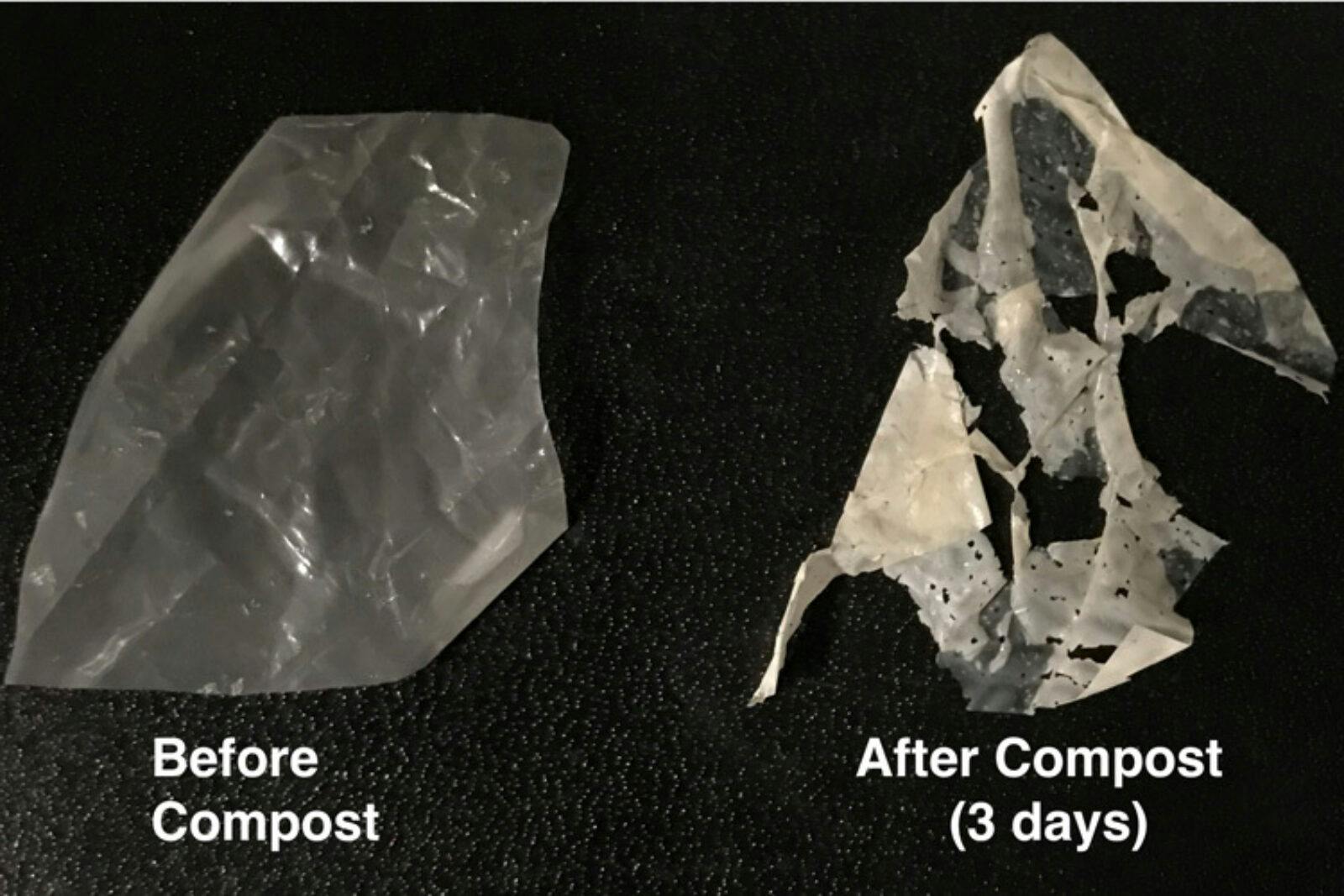
The Author
The Researcher
Here is a surprising fact about "biodegradable" plastics: They take a long, long time to fully decompose. That makes them impossible to compost with food and other organic materials. But a new technology promises to solve this problem. Developed by Ting Xu, a member of the Kavli Energy NanoScience Institute at UC Berkeley, where she is a professor of chemistry and materials science, it promises to break plastics down into molecules that renew and replenish soil bacteria, help decontaminate toxic spills, and change the way we make medical implants.
When biodegradable plastics were introduced decades ago, they seemed to check off all the right boxes. They are made from naturally occurring materials that microbes can eat. And they seemed to disappear when dropped along roadsides or buried in landfills.
But they never fully broke down into something that was recyclable outside of a test tube. This is due to how they were made, which involves taking naturally occurring chemicals and synthesizing them into long polymer chains. Over time, those chains break apart leaving behind tiny particles of the same material called microplastics.
Microplastics—particles 5 millimeters in length or smaller—make their way into the soil, water, and air, where they can wind up in the bodies of fish, animals, and people—with as-yet unknown effects.
Xu’s approach turns to enzymes, proteins that catalyze chemical reactions in living things, to break down polymer chains. She has developed a way to encapsulate enzymes within plastics. When triggered by modest amounts of heat and water, the enzymes begin gobbling up polymer chains, leaving behind only small molecules that serve as food for soil bacteria.
This is the first time anyone has developed a way to embed enzymes nanoscopically in plastics with such high levels of activity. The approach is highly effective and uses readily available commercial materials and techniques, which also makes it highly affordable.
“If you want to see the enzymes we are using, just eat some low-fat yogurt,” she said.
Polymer science

Xu came to biodegradable polymers by an unusual path that started with polymer science bachelor’s and master’s degrees in China, followed by a Ph.D. from University of Massachusetts, Amherst, in 2004.
Then she switched gears. During a three-year post-doctoral fellowship at University of Pennsylvania and NIST, Xu studied how to apply what she had learned about polymers to large biomolecules. Her goal was to synthesize proteins not found in nature and that could perform unusual tasks from scratch.
“When I looked at fields like tissue engineering, drug delivery, and regenerative medicine, it was clear researchers were looking at them only from the vantage point of biology,” Xu said. “The field lacked a deep molecular understanding of polymers, and that’s where I thought I could help.”
It required a wholly new mindset. At first, Xu struggled with the terminology, especially “reading” the amino acid building blocks of proteins. “The other people in the lab could just glance at a sequence of amino acids and say, ‘That’s where you should look,’ or ‘That’s an alpha helix,’ or ‘This one will have a binding site.’ I didn’t see any of that.”
Within six months, though, she began to see similarities between biomolecules and the synthetic polymers she had worked with before. She likens both to beaded Mardi Gras necklaces. In polymers, the beads were only one color because they are made of repeating chemical units, while in proteins the beads are 20 different colors, one color for each of the 20 amino acids found in proteins.
Xu wanted to weave those two necklaces together, but bonding proteins and polymers destroys the ability of proteins behave like proteins. This is because proteins are shapeshifters, Xu explained. While they, like polymers, consist of a long strand of atoms, they fold into a variety of complex configurations, each with its own unique chemical reactivity and behavior.
This propelled Xu on a quest to understand how polymers and proteins respond when they get close to one another. It took seven years to map the energy landscape of those interactions, carefully measuring when a polymer would denature a protein or when a protein would rearrange a polymer.
Then she made a bold assumption that enabled her to discover a way to keep proteins safe and secure when embedded in plastics.
Cradles
That assumption started with a question. When Xu looked at our bodies, she saw proteins everywhere, from inside our cells and bloodstream to the fluid that lubricates our knees. Why didn’t these proteins react with one another uncontrollably?
So, she made an assumption: “There had to be some sort of molecule—maybe a long-chain sugar or a protein—that chaperones these proteins around,” Xu said. It has to bond strongly enough to keep them from interacting randomly, but not strongly enough to keep them from changing their shape and going into action when they are needed.”
Nature, she assumed, had to have some universal rule for building such loosely binding materials. She set out to uncover it by analyzing the protein-polymer interaction data she had accumulated over the past seven years and the protein sequences she had studied as a graduate student.
The universal rule proved surprisingly simple: It involved the average length of the amino acid sequences that determined whether a protein was attracted or repelled by water. Using that rule, she could build molecules that would and neutralize and protect proteins without taking away their ability to shift shape.
Xu worked with a class of materials called random heteropolymers. It consisted of four subunits, each designed to interact with a different segment of any natural folded protein’s surface.
In 2018, she showed how this could work by encapsulating enzymes that break down phosphorus-based chemicals in a random heteropolymer. She then embedded them in a plastic matt. Within minutes of submersing the mat in a basin of insecticide, the enzymes had broken down 10 percent of the toxic organophosphate active ingredients.
It was the first time anyone had incorporated an enzyme that retained a majority of its activity in plastic. “One reason we could do it and others couldn’t is that we had learned how to stabilize those enzymes,” Xu said.
Composting
Xu then turned her attention to biodegradable plastics. First, she chose two popular compostable polyesters, polylactic acid and polycaprolactone, which are made from corn starch or sugar cane. Then she identified enzymes that could chew up those long polyester chains, leaving behind only small molecules of lactic acid or caprolactone, which soil bacteria eat as food.
Xu needed to protect the enzyme from the high temperatures used to melt polyesters during processing. Polylactic acid, for example, melts at 338** F (170** C), more than hot enough to pull apart most enzymes.
Cradling it in a random heteropolymer kept that from happening. The cradle let the enzyme stretch out and change its shape as it heated. The enzyme could even lose some of its hydrogen bonds, but it would regain them as it cooled. As long as the cradle confined the enzyme, the heat would not pull it apart.
Xu also needed to position the enzymes in the right location. Enzymes, it turns out, can either randomly break long biodegradable polymer chains into shorter pieces or devour them the way we eat hot dogs—starting from one end and working their way towards the other.
Fortunately, polymer chemistry made this easy. Polymers have very uniform chemical properties along their length, which enables them to align as crystals when they solidify. On their tails, the properties are less uniform. As a result, polymer tails are pushed out from the crystalline regions and into amorphous areas of the plastic.
The chemical properties of cradled enzymes push them into those same amorphous areas. This positions them where to go to work. Once they are exposed to heat and water, the enzymes slip free of the cradle and begin attacking the polymers.
It does not take a lot of enzymes to do the job, only 0.02 percent by weight for polylactic acid and 0.05 percent by weight for polycaprolactone. These are added to the polymers before they are melted. The amount is so small, it changes neither the polymers’ processing characteristics nor its performance.
Yet these small additions are highly effective. After adding water, 80 percent of the polylactic acid degraded into lactic acid molecules within a week. Under industrial composting conditions, which featured moderately higher temperatures, it all disappeared within six days. Composted polycaprolactone was gone in only two days.
Commercial possibilities
Xu believes cradled enzymes could be economical enough to use commercially. Part of the reason is that it is built on existing technologies.
“We do nothing new,” she said. “There’s no new chemistry. There’s no new polymer, just the biodegradable plastics we are already using. There’s no new enzyme, just commercial grade enzymes that people already make. The cradle is cheap, made of readily available methacrylates. The chemistry automatically positions them where they need to go.”
Yet the way Xu put all these components together could change how we recycle. Right now, recycling is limited to larger objects, like plastic milk bottles and food containers. Hardly anyone thinks about recycling candy wrappers or the smaller plastic containers they pick up from storefronts on the way to work or at lunch. Those plastics often wind up in landfills. If they contained cradled enzymes, they would degrade completely.
Yet biodegradable plastics are only one of many potential uses for active enzymes incorporated into plastics. The U.S. Army Research Office, which funded some of Xu’s work, believes the materials have potential for decontamination, sensing, and self-healing materials.
Xu thinks they may one day go into the glues that hold together computer circuits and smartphones. When a product is obsolete, the glue would dissolve, and the parts could be recycled.
Xu is even more excited about biomedical uses —because her cradles can hold more than enzymes. In fact, they can deliver medicines.
Today, implants provide controlled release medicines by breaking down over time. Yet their breakdown is neither fully controlled nor fully understood, Xu said. They could leave behind microplastics that could potentially clog smaller blood vessels or evoke an immune system response.
Enzyme-embedded plastics would solve some of those problems. They offer a highly controlled way to release medications while ensuring that only small and easily eliminated molecules enter the bloodstream. Such materials could also be used for scaffolds to prompt tissue growth and for threads and staples for closing surgical incisions. They could also deliver other proteins, such as insulin.
The possibilities are almost endless, and biodegradable plastics are just the beginning.