Astronomically Seeing is Believing
by Adam Hadhazy
How the first-ever image of the monster black hole lurking in the heart of the Milky Way dispels any lingering uncertainty about the previously unseen object's nature
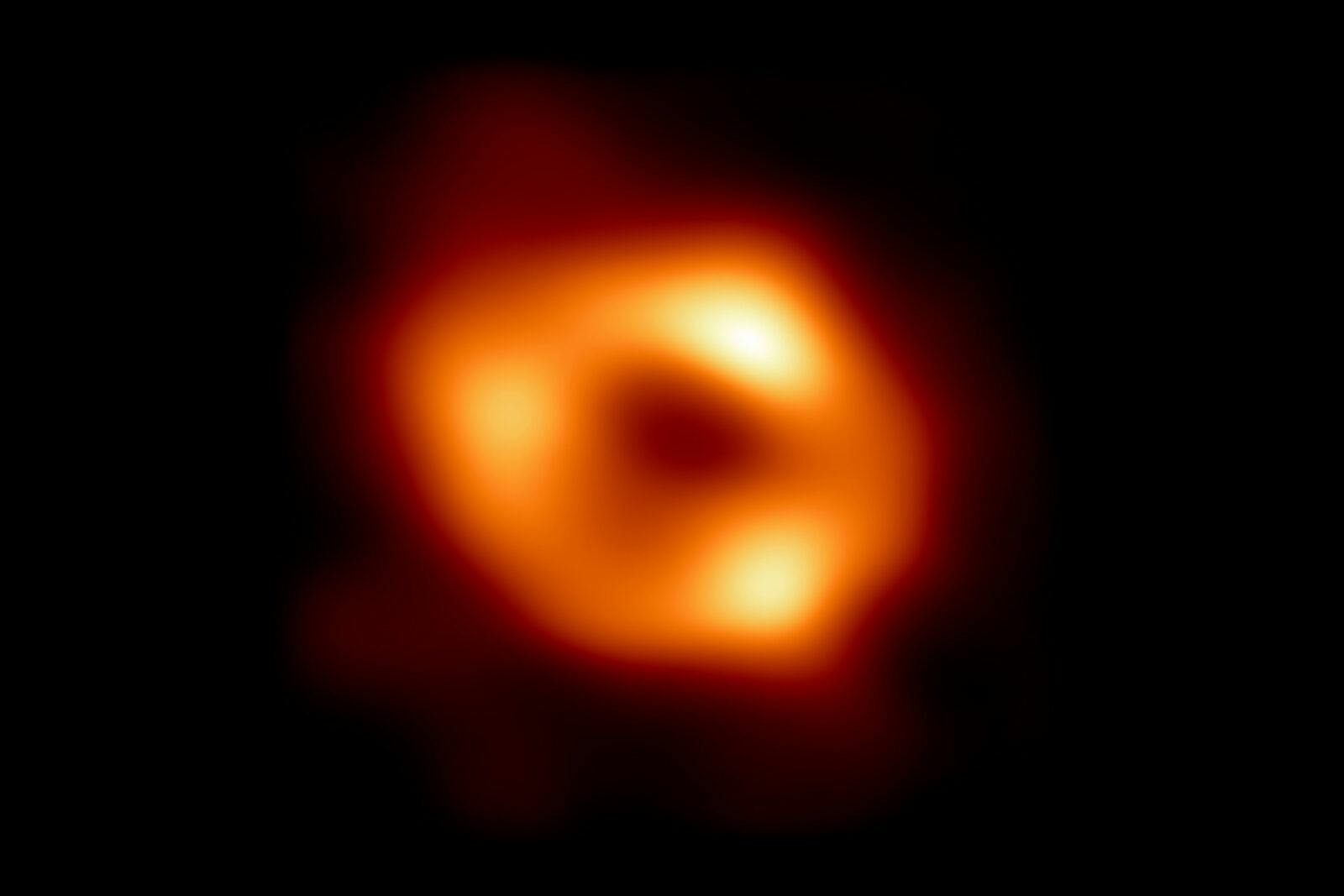
The Author
Extremely little doubt existed in the scientific community that the distinct radiation source in the apparent center of our Milky Way Galaxy was anything but a black hole. Although we had lacked the ability to see it directly, multiple lines of evidence built up a strong case. Highly convincingly, stars near this object—dubbed Sagittarius A*, or "sadge-ay-star"—move in dramatically fast, tight orbits. Extrapolating from these orbital motions leads to the conclusion that the gravity from a supermassive, compact object is dictating these orbital maneuvers. Additional supporting information includes the observation that objects fitting the theoretical descriptions of black holes are found at the center of so many other galaxies. Yet despite the overwhelming evidence, in this matter as well as many others, seeing is still believing. So it has come as a powerful validation—with admittedly a dash of relief mixed in—that a revolutionary global experiment designed to directly image Sagittarius A* has found the object to be just what we thought it was. The international experiment, the Event Horizon Telescope (EHT), has the involvement of many members of Kavli astrophysics institutes. The reveal of Sagittarius A*, which took place last month, is a triumph, both for sophisticated science and also for the base, undeniable animal desire to plainly behold a thing to accept it as real.
The first-ever image of the Milky Way's central supermassive black hole
Details-wise, the EHT is a globe-spanning network of radio telescopes, from the South Pole to Greenland. Each observatory in the network is paired to form baselines of varying geographical lengths. Each baseline can capture certain frequencies of radio waves from Sagittarius A*. Combining those observations, along with intricate data analysis, allows for an overall image of the object to be arrived at, and with stunning resolution; seeing Sagittarius A* is like being able to visualize a donut on the moon. The donut analogy is further accurate in the sense that the image captured by EHT is of a ring of light-emitting matter just outside of the event horizon of Sagittarius A*—the boundary at which a black hole's gravity becomes so strong that not even light can escape. A huge team of researchers, from experimentalists to theorists, had roles to play in delivering the results. Researchers at the Massachusetts Institute of Technology's Kavli Institute for Astrophysics and Space Research, the Kavi Institute for Cosmological Physics at the University of Chicago, and the Kavli Institute for Astronomy & Astrophysics at Peking University were just some of those involved. The observations themselves took place in 2017, alongside observations of a farther-away, but significantly bigger black hole at the heart of the galaxy M87; those breakthrough results were announced in 2019. The Milky Way's black hole proved harder to image, both because of its location through the obscuring disk of our galaxy, and also because Sagittarius A* is more variable in appearance than M87's black hole. Further study of black holes by EHT will deepen our understanding of black holes as well as test fundamental laws of physics
Wielding the sun's gravity as a zoom lens for exoplanets
As telescope ideas go, it's a wild one. In 1979, the late Stanford University professor Von Eshleman showed that placing a space telescope at a special focal point at the edge of the solar system could capture images of far-off objects magnified and distorted by the sun's gravity. Researchers have since developed the concept further, realizing that such a solar gravitational lens could enable the detailed study of exoplanets. The magnification power harnessed would reveal worlds' atmosphere, colors, continental shapes, and surface features such as forests or even cities. One challenge, though, is that image would be highly distorted and span a focal region of space about a kilometer in diameter—far too large to build a detector surface across. One solution would be to have a telescope (or scopes) move around to gather observations from location after location in this focal expanse, like gathering up pixels to form a whole picture. Movement, though, means fuel or an energy source, which adds to mission complexity and represents a potential point of failure. In a recent study, two researchers at the Kavli Institute for Particle Astrophysics and Cosmology explored how using algorithms could in effect reverse the bending of the light by the sun and deliver an undistorted image. Combining this technique with the pixel-gathering approach could combine for the best resolution and offer us unprecedented views of alien worlds, likely many decades hence when such a mission could be feasibly executed.
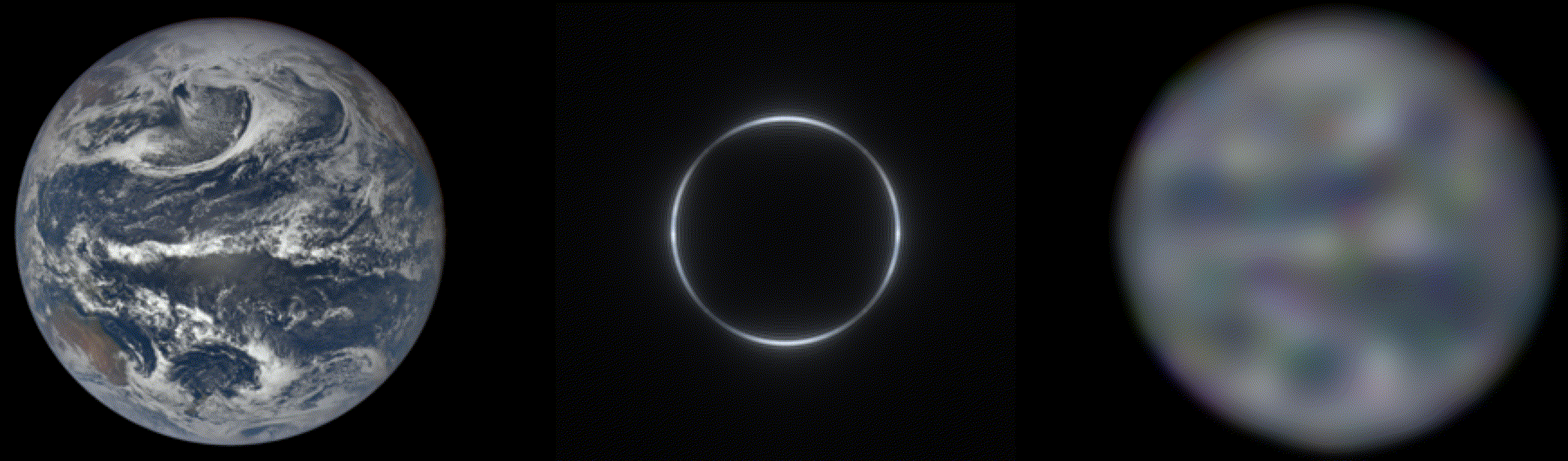
Seeing back to the progenitors of the universe's supermassive black holes
In a blog post from NASA about the recently launched next-generation James Webb Space Telescope, Roberto Maiolino, former director of the Kavli Institute for Cosmology, Cambridge explains how Webb will help in the search for primeval black holes. These mysterious, theoretical objects served as the "seeds" of supermassive black holes. Those monster black holes are found in the modern universe at the hearts of galaxies—for instance, right here as Sagittarius A* in the Milky Way. But how exactly these objects ever got so big in the first place is a major question. Maiolino explains that Webb will be able to see back to extremely early galaxies with actively growing black holes in them. Studying these black holes should help resolve whether such objects arose from the collapses of the first, ultra-hefty stars, or from the direct collapse of colossal gas clouds, or from the merging of many smaller black holes within dense star clusters.
Honing the hunt for magnetic monopoles
Magnetic monopoles have long fascinated—and frustrated—physicists. These hypothetical particles consist of a single magnetic pole or charge, equivalent to how other particles can have either a positive or negative electric charge. Extensive searches to date, though, have yielded no evidence of the flummoxing particles. In a new study, researchers at the Kavli Institute for the Physics and Mathematics of the Universe have pulled together data from artificial, human-made particle colliders to guide the continuing search using natural particle colliders as a search method. Those natural particle colliders come in the form of fast-moving atomic nuclei and other particles, called cosmic rays, that smash into Earth's atmosphere. By setting some boundaries on the energies that magnetic monopoles generated by cosmic rays could possess, researchers hope to narrow the search and thus look in the likelier places. Finally nabbing a magnetic monopole would deliver key new insights into—as the Kavli Institute's name says—the physics and mathematics of the universe.
"Echoing" black holes offer insight into galactic-scale star formation stoppages
The gravity from colossal black holes at the centers of galaxies can whip up nearby matter to extreme speeds and energies, producing outflows of radiation that can amazingly halt new star formation throughout whole galaxies. It's a far-reaching phenomenon researchers would like to know more about. And now thanks to some discoveries of smaller black holes closer to home, here in our Milky Way, researchers have more to work with. Researchers at MIT's Kavli Institute for Astrophysics and Space Research led a team that has quintupled the number of known "echoing" black holes, jumping from two to 10. The echo results as emissions from the hot plasma region, called the corona, just outside the boundary of the black hole reverberate off the disk of material swirling around the black hole. Using the NICER instrument aboard the International Space Station and an algorithm dubbed the Reverberation Machine, the researchers isolated the new echoing black holes and studied them. The researchers noted that the corona can undergo an evolution from a high-energy to low-energy state, giving off a final blast of radiation during the transition; that final blast may be the same star formation-killing burst given off by these black holes' far larger cousins in galactic cores.
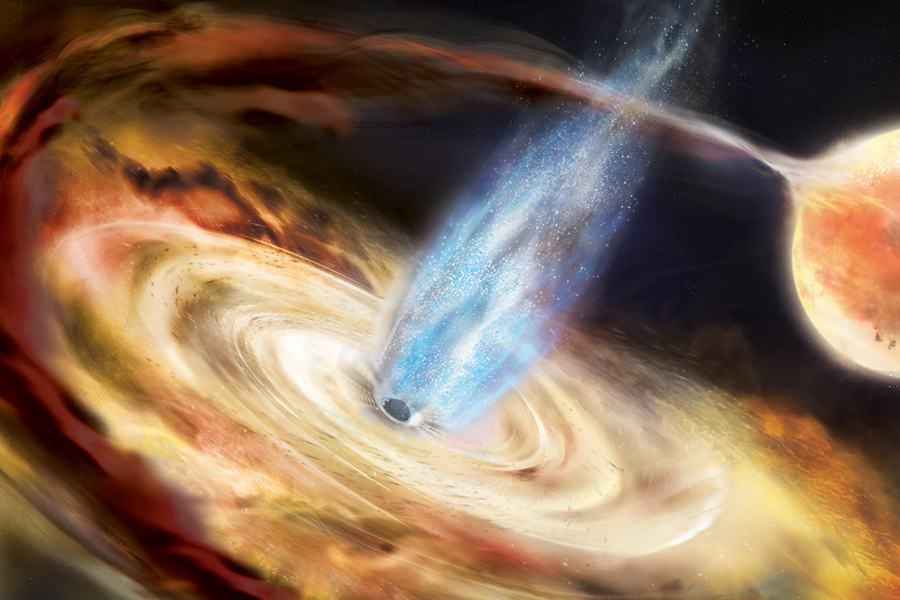