The Search for Dark Matter (Sidebar)
Key details on dark matter
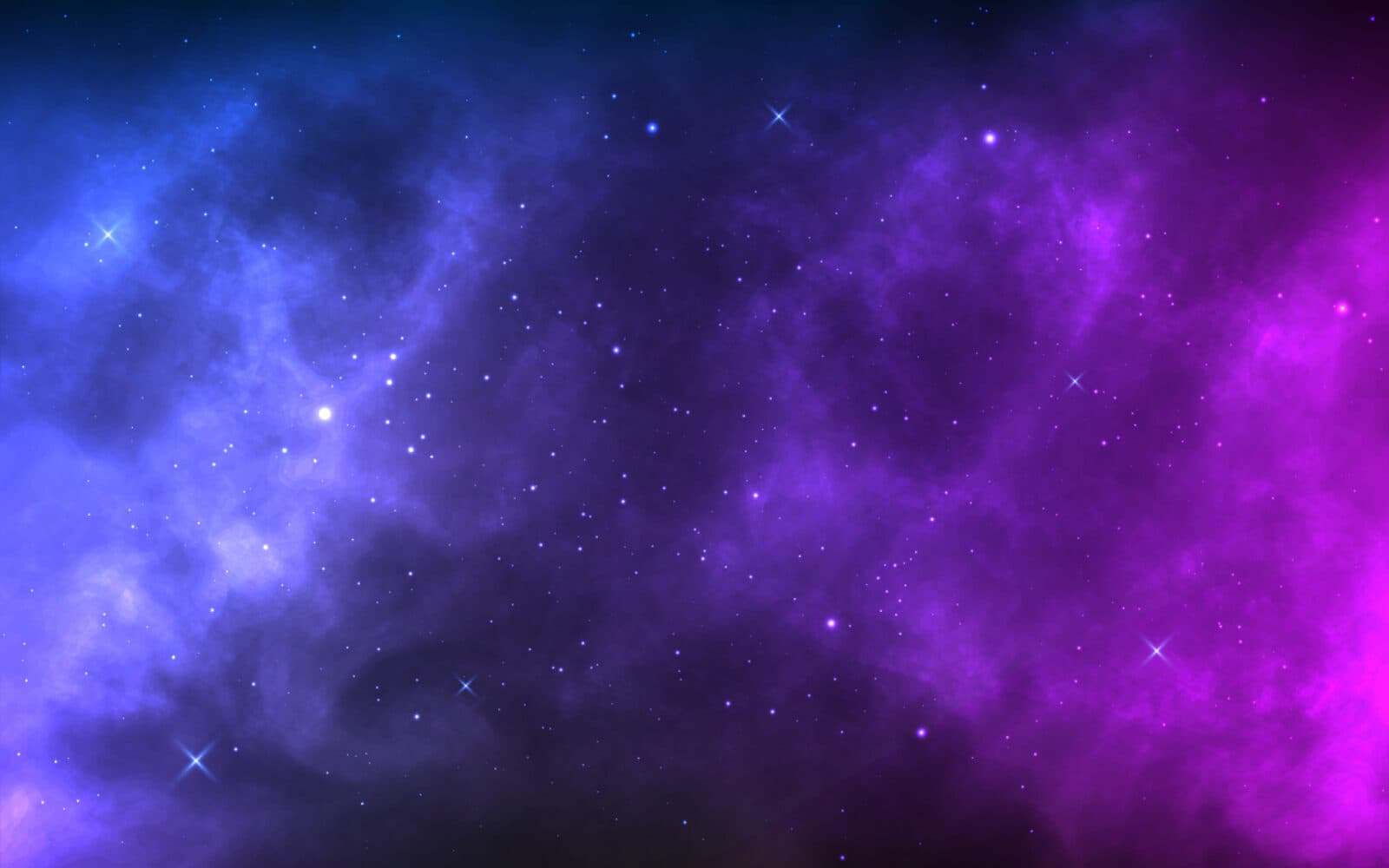

DARK MATTER WAS FIRST PROPOSED in 1933 by Swiss-American astronomer Fritz Zwicky, who observed that the gravitational mass calculated for the Coma galaxy cluster was at least 400 times what he expected by observing the cluster’s luminosity. The Coma cluster, which has more than 1,000 galaxies with a mean distance of 321 million light years from Earth, is in the direction of the Northern sky constellation Coma Berenices, near Leo.
In the following decades, more precise measurements of the velocity of stars at the outskirts of galaxies, by American astronomer Vera Rubin and others, solidified the idea of dark matter. Something else besides stars, planets, gas, and other ordinary matter is giving galaxies – and galaxy clusters, for that matter – the extra mass they need to hold themselves together.
But what is dark matter? We don’t know, and that’s why physicists call it “dark.” Unlike ordinary matter, it doesn’t emit light or any other kind of radiation. So far it has been studied observationally only through its gravitational effects on ordinary matter. At the same time, dark matter helps physicists explain their overall physical model of the universe.
There are some ideas for what dark matter might be, and cosmologists, astrophysicists and experimental particle physicists say they are closing in on an answer. One leading candidate is the WIMP, or Weakly Interacting Massive Particle. A sea of WIMPs, under this idea, is thought to permeate space. Hardly “massive” by any common definition, WIMPs are nevertheless expected to be a kind of massive fundamental particle. Over more than half a century, particle physicists have reduced nature to progressively tiny and exotic fundamental particles that make up atoms and the forces that govern them. We’ve long known that the basic constituents of nature are far more numerous and varied than the protons, neutrons and electrons that many people first learned about in high school. The WIMP may fit into this enlarged family of fundamental particles, just as the tentative discovery of the Higgs boson particle helps explain what gives ordinary matter mass.
Researchers are working on three primary fronts to learn about dark matter. One way is by measuring it directly in staggeringly sensitive detectors that record the rare collision of a dark matter particle with an atom of germanium crystal, liquid xenon or another substance.
A second way is to indirectly observe the expected products of WIMP collisions in space. The Fermi Gamma-ray Space Telescope, launched in 2008, is searching for one such product, gamma rays, which are thought to be produced when dark matter particles collide and annihilate in the galactic halo, a spherical region surrounding the Milky Way Galaxy. Another indirect detection instrument, called the South Pole Neutrino Observatory, or IceCube, is searching for a sub-atomic particle called a neutrino. Neutrinos are believed to be created when WIMPs pass through the sun and interact with solar protons and helium nuclei.
The third way physicists are searching for dark matter particles is with particle accelerators such as the Large Hadron Collider at CERN, the European Organization for Nuclear Research near Geneva, Switzerland. Particle physicists have hypothesized that dark matter particles may reside in a kind of shadow world of “force carrier” particles that mirror the fundamental “matter” particles, such as electrons, quarks and neutrinos, that physicists have discovered. This idea, called supersymmetry, could mean that dark matter particles remain hidden from view. However, if fundamental particles are crashed together at sufficient energies, supersymmetry particles may be revealed – and among them may be WIMPs.