Big Name, Big Game from the Square Kilometer Array
by Adam Hadhazy
Past and present members of the Kavli Institute for Cosmology, Cambridge, gear up the science for the largest radio telescope ever built
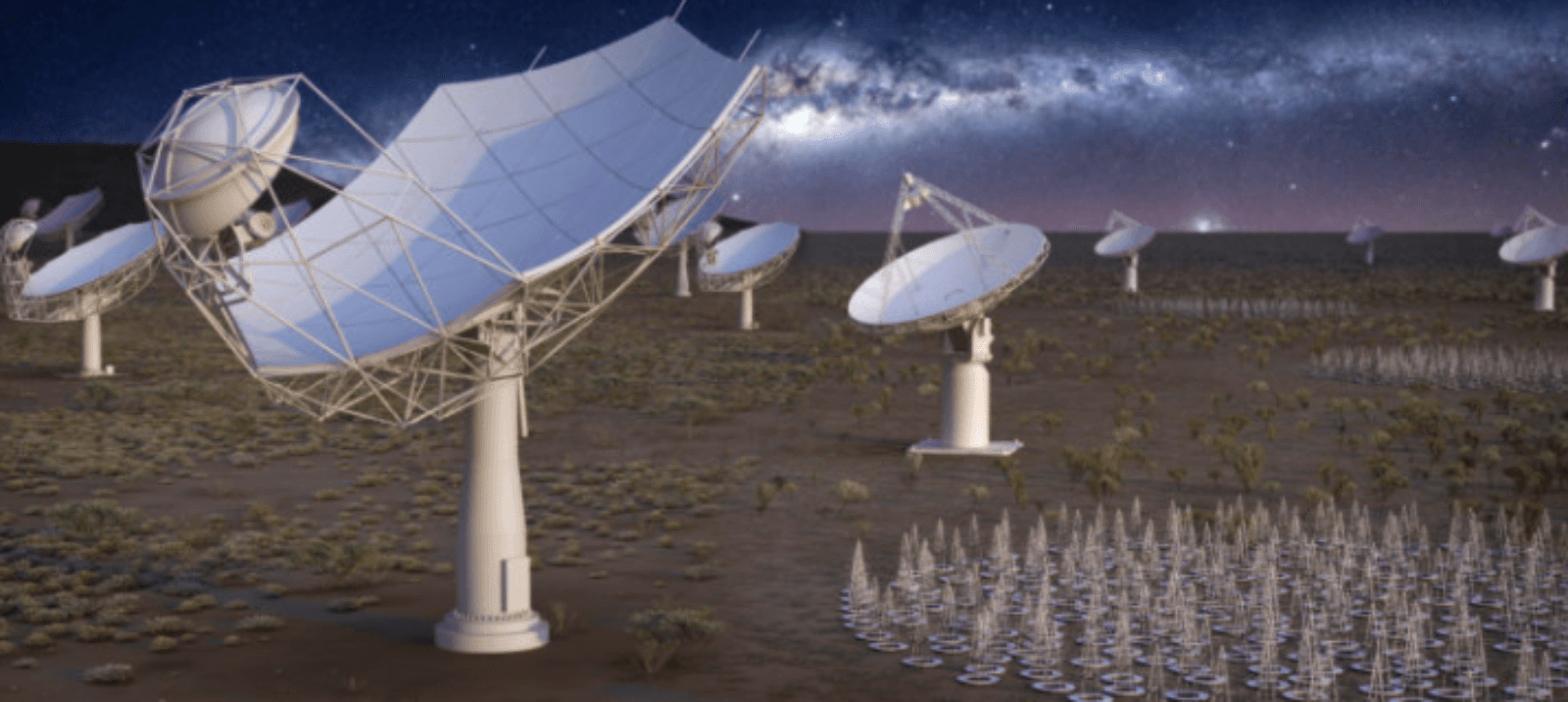
The Author
The Researchers
Sometimes, the ambitious scale of a scientific endeavor is right there in the name. So it is with the Square Kilometer Array, or SKA, shaping up as the world's largest and most powerful radio telescope. If all of its oodles of antennas are eventually built, it would have a collecting area of one million square meters—a record-setting square kilometer, that is.
The project has been in the works for nearly 30 years and has had its fair share of hurdles to overcome, budgetary and otherwise. Yet for now, the prospects look good for building out the first iteration of SKA, dubbed SKA1. This array would bring to bear about 10% of the proposed final array size (designated SKA2). SKA1 is scheduled to and come online for science observations in the mid-2020s with the full, SKA2 array slated for the 2030s.
SKA would ultimately take radio signals received by thousands of dishes, coupled with as many as a million low-frequency antennas, spread across two remote, pristine sites in the Karoo region of South Africa and the Murchison Shire in Western Australia.
As might be expected for such a generational, trans-continental instrument, the science returns from SKA would be massive—even during its early, smaller-scale implementation. "The SKA1 telescopes will provide a huge step forward in our understanding of some of the fundamental properties of the universe," says Jeff Wagg, a project scientist at the SKA headquarters in Jodrell Bank, UK, and a former member of the Kavli Institute for Cosmology, Cambridge (KICC) at the University of Cambridge.
Other current KICC researchers are contributing to the cause, including Anastasia Fialkov. She is a core member of a group examining how SKA can delve into the Epoch of Reionization—a major era in cosmic history when the universe went from being a dark and largely featureless place to a universe like today, full of stars and galaxies. The Epoch, which started roughly 400 million years after the Big Bang, gets its gnarly name from the changing state of the sea of neutral hydrogen atoms then permeating the universe. Powerful light from the first stars energized this neutral hydrogen gas, ionizing it (knocking an electron out of, technically). In time, all the once-neutral, gaseous hydrogen had turned into a state of matter, called plasma, that has suffused the cosmos since.
Fialkov is modeling the theoretical cosmological signal produced by those neutral hydrogen atoms in the early universe. Due to the expansion of the universe, this electromagnetic signal gets stretched out into the radio wave frequency band that SKA detects. "Once detected, this signal can tell the story of our baby universe," says Fialkov. "We can learn about the nature of the very first stars and black holes."
Other big-ticket science goals for SKA researchers include wielding the array to perform extreme new tests of general relativity, Albert Einstein's astonishingly enduring theory of gravity. SKA would do so by possessing the sensitivity to precisely measure variations in the timing of fascinating objects known as pulsars. The leftover, ultra-dense cores of colossal stars, pulsars spin up to hundreds of times per second, sweeping a beam of radiation through the cosmos like a crazed lighthouse. Variations in this timing can occur when the interwoven fabric of space and time itself, called spacetime, is sent a-rippling, much like the surface of a pond, by a phenomenon known as gravitational waves. In the last several years, these ripples—first predicted by Einstein over a century ago—have been detected from the mergers of black holes and neutron stars. But a different kind of gravitational wave is expected to rip forth from the collisions of supermassive black holes. Pulsar signals should prove an optimal means of detection of these waves.
As Wagg says, "with SKA1-MID"—referring to the middle-frequency range of the instrument—"we will be able to time millisecond pulsars to a level of accuracy that will be sufficient to reveal direct evidence for the nanohertz gravitational wave background produced by the mergers of supermassive black holes."
Still other science goals will involve mapping cosmic evolution across the eons, discerning the role of poorly understood cosmic-scale magnetic fields, and even aiding in the search for extraterrestrial life via radio signals an intelligent alien civilization might be spilling into space.
Lest all that sound awfully ambitious, remember: It's right there in SKA's name.