Black Holes Bear Astrophysical Fruit
by Adam Hadhazy
Interesting enough in their own right, black holes are additionally of great benefit to scientists as means to test out theories about the laws of physics beyond just gravity
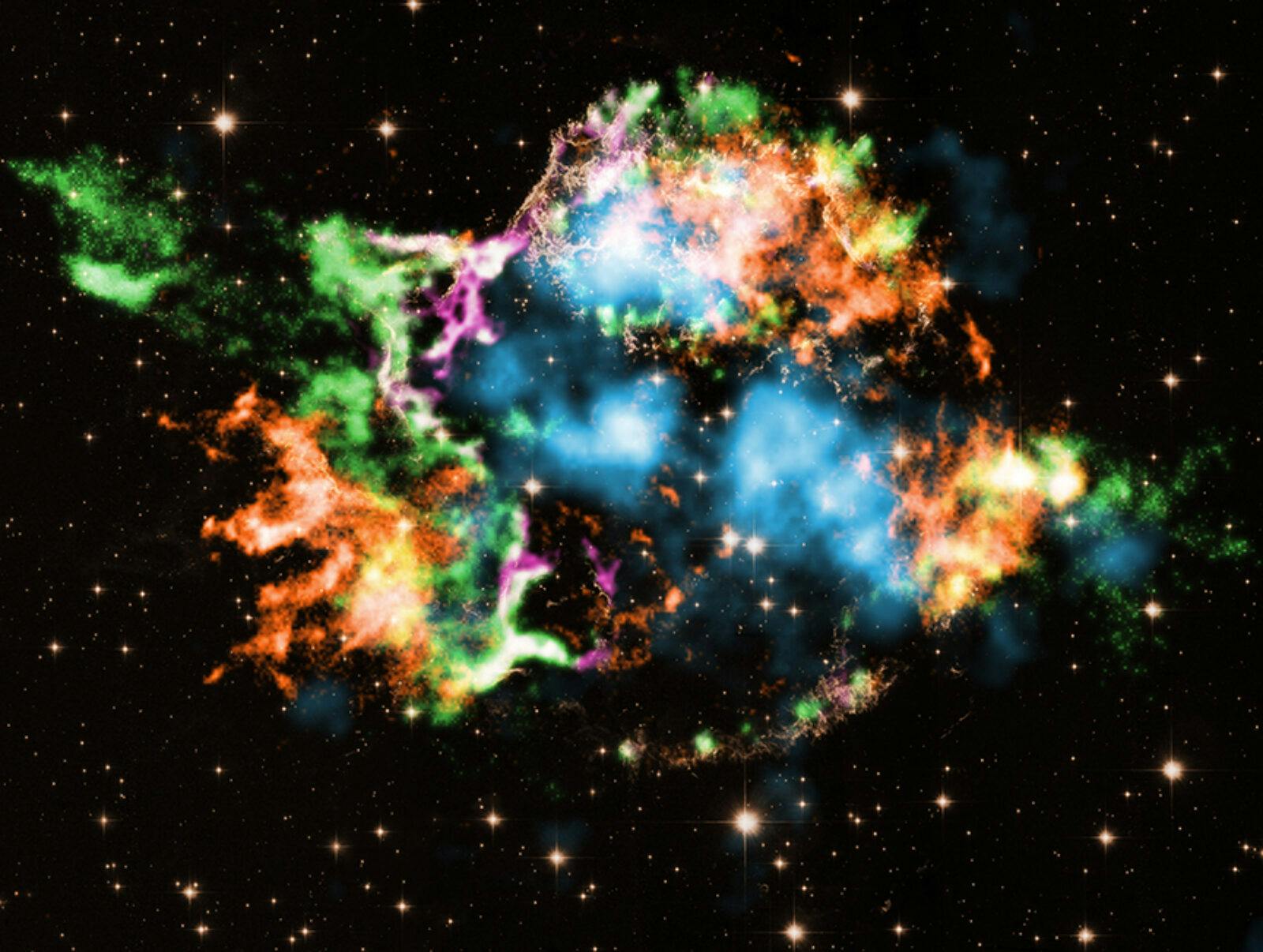
The Author
Despite black holes' intrinsic nature and namesake of being invisible, these objects sure are proving handy as astrophysical measurement devices. For although black holes' intense gravity indeed prevents the escape of light once light (or anything else) strays too close, the environment immediately beyond this point of no return, the so-called event horizon, can teem with observables. To wit: going back to the initial tentative discovery of black holes almost 60 years ago, the way we've historically detected these beasts is via the immense amounts of energy beamed out by matter captured in a black hole's grip. As the gravity of black holes accelerates matter surrounding the black hole to incredible velocities, the matter whips around and smashes together and unleashes light across the electromagnetic spectrum. That's for starters. Since 2015 and the debut of gravitational wave detectors, researchers can now also study black holes via the ripples they generate in the spacetime fabric of the universe. Overall, this means that with the electromagnetic radiation streaming from the vicinity of black holes as well as gravitational radiation, researchers can leverage the extreme properties of black holes to test the fundamental workings of the universe itself. These investigations have long included tests of Albert Einstein's famous general relativity, our powerfully prevailing explanation of how gravity manifests. Just this past month though, Kavli Institute-affiliated researchers have proposed new ways of wielding black holes' effects to test more speculative topics. For instance, black hole spin rates have now been recruited in the search for dark matter. Someday, we might be able to employ black holes in even more exotic quests, like validating string theory. It seems that as black holes consume some admittedly tiny bits of our universe, the objects not only taketh, but also giveth.
Spinning black holes rule out a narrow range of dark matter particle masses
An innovative way to search for dark matter particles via measuring the spin of black holes has been demonstrated by researchers at the Massachusetts Institute of Technology’s Kavli Institute for Astrophysics and Space Research (MKI). According to theory, a type of dark matter candidate particle known as an ultralight boson should form en masse in the vicinity of a black hole. If so, these particles would slow down the black hole's spin. Thanks to LIGO and other observatories, in the last six years scientists have gained the ability to measure black holes' spins because the gravitational waves generated when black holes collide carry a signature of the objects' spin. When looking over the catalog of black hole spins recorded to date, however, the researchers found some with spins far too fast for ultralight bosons to have affected the black holes, ruling out a certain mass range of the particle. But because the particles can have a vast range of masses, the search is very much still on, and black holes can continue in helping rule out—or, just maybe, just in—other possible masses.
New way for black holes to act as a testbed for string theory
Members of the Kavli Institute for the Physics and Mathematics of the Universe (Kavli IPMU) have written an intriguing paper on how powerful observatories should be able to use black holes to test string theory. String theory is a beautifully elaborate mathematical "theory of everything" that seeks to unite all four fundamental forces of nature, positing every particle and force it conveys as an excitation of a miniscule, string-like entity. The theory is as breathtaking as it is frustrating, however, seeing as it is notoriously difficult to subject it to testing for validation. Yet in the new paper, string theory is shown to resolve a problem identified by the researchers wherein singularities—hypothetical points where density is infinite—form multiple times over when a photon travels around a black hole. The curved spacetime (manifesting as gravity) around a black hole would stretch strings out, preventing such singularities. The effect is too small to currently observe, but highly powerful instruments down the road could facilitate such a test.
Honing data to quicken the hunt for dark energy
Progress in nailing down the properties of dark energy has been announced by researchers at the Kavli Institute for Particle Astrophysics and Cosmology (KIPAC) at Stanford University. The scientists are working with data from the Dark Energy Survey (DES), a project that gathered deep observations of a slice of the sky over a six-year stretch (and that has heavy involvement from researchers at the Kavli Institute for Cosmological Physics at the University of Chicago). DES hoovered up measurements on the distribution of matter, galaxies, and galaxy clusters in its representative observed slice of the cosmos. Troublesomely, these three quantities are closely interrelated, such that errors can easily arise and skew results. The researchers developed a new analysis method that accounts for these connections, enabling all three quantities to be considered together in ultimately delivering more precise estimates of matter's density and clumpiness on grand scales. Figuring these measurables out further will help in tracking how the universe's acceleration has expanded, courtesy of dark energy, and accordingly place limits on dark energy's nature.
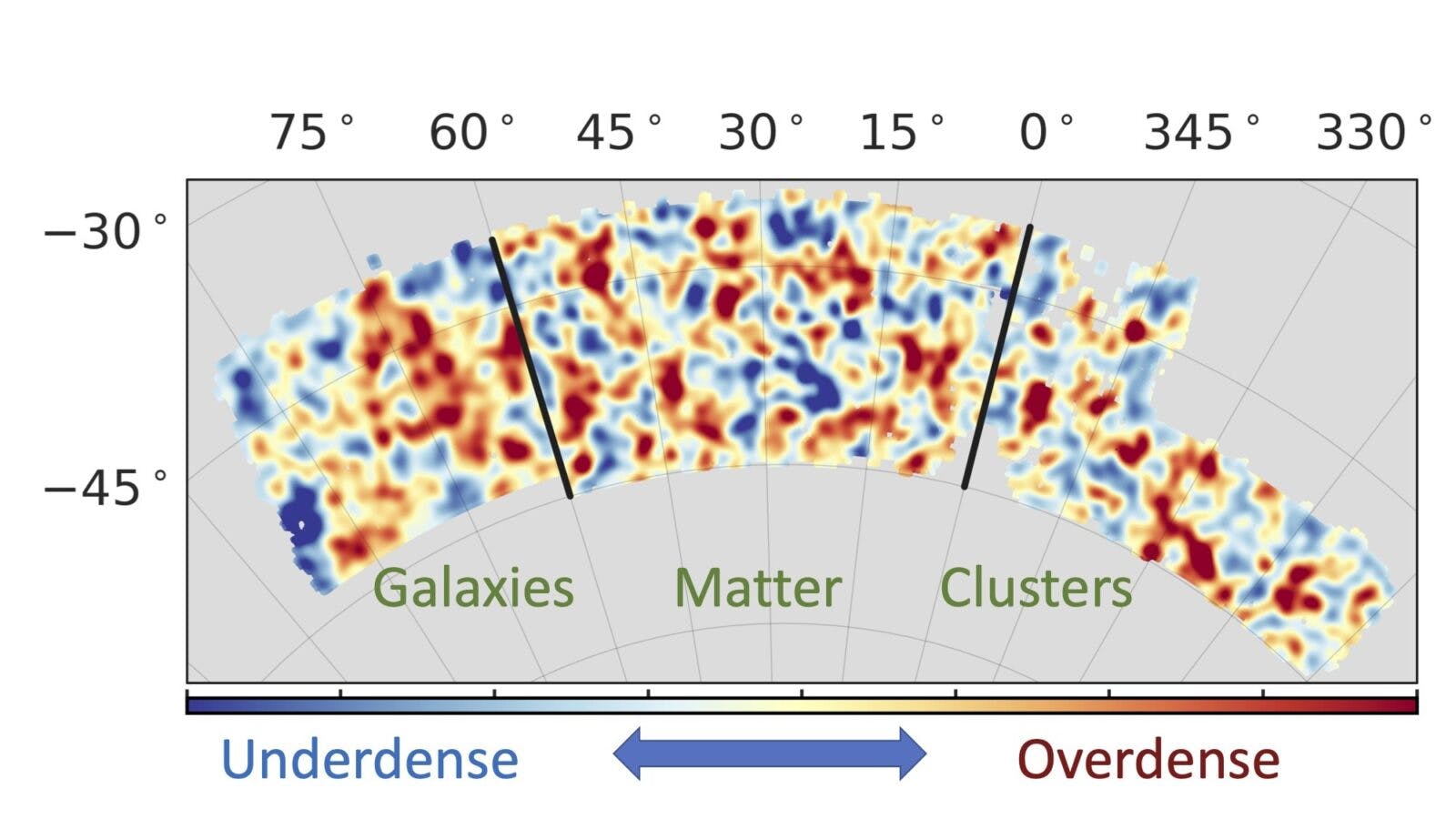
Titanium detection supports neutrino-driven model of supernovae explosions
Researchers at Kavli IPMU were involved in a recent discovery of stable titanium in a supernova explosion made by the Chandra X-ray Observatory. Previously only radioactive titanium had been glimpsed in these mammoth stellar explosions. The finding of stable titanium comports with theories on how stars with 10 times or more mass than the Sun collapse and go boom. While the broad outlines of supernovae are fairly well-understood, many critical details have yet to be verified. For example, extremely lightweight, vet gobsmackingly numerous particles called neutrinos are thought to help drive the stellar explosions by producing high-speed bubbles. The new findings buttress models incorporating neutrinos for at least some of these explosions, suggesting researchers are on the right track.
Developing gamma-ray burst-based methods for measuring cosmic distances
KIPAC researchers and colleagues continue to make advances in wielding super-luminous events known as gamma-ray bursts as precise tools for handily measuring cosmic distances. The challenge involves the fact that GRBs, as they're known for short, vary across a range of observable properties, such as duration and brightness, and which likely relate back to a range of GRB-producing star system properties. The trick, then, is to find a way to make apples-to-apples comparisons, allowing of course for the fact that the apples will vary in sizes and shapes, so to speak. The researchers are thus examining observable features of GRBs that would facilitate these standardized comparisons in the x-ray and optical bands, seeking connections between luminosity and changes in luminosity over time. It's still a work in progress, but if borne out, cosmologists would have one more way to independently check cosmic distances, important for understanding the universe's evolution and fate.