Boosting Neuronal Resilience
by Sally Johnson
Graeme Davis wants to maximize the self-corrective powers within everyone’s brain to resist neurodegenerative diseases
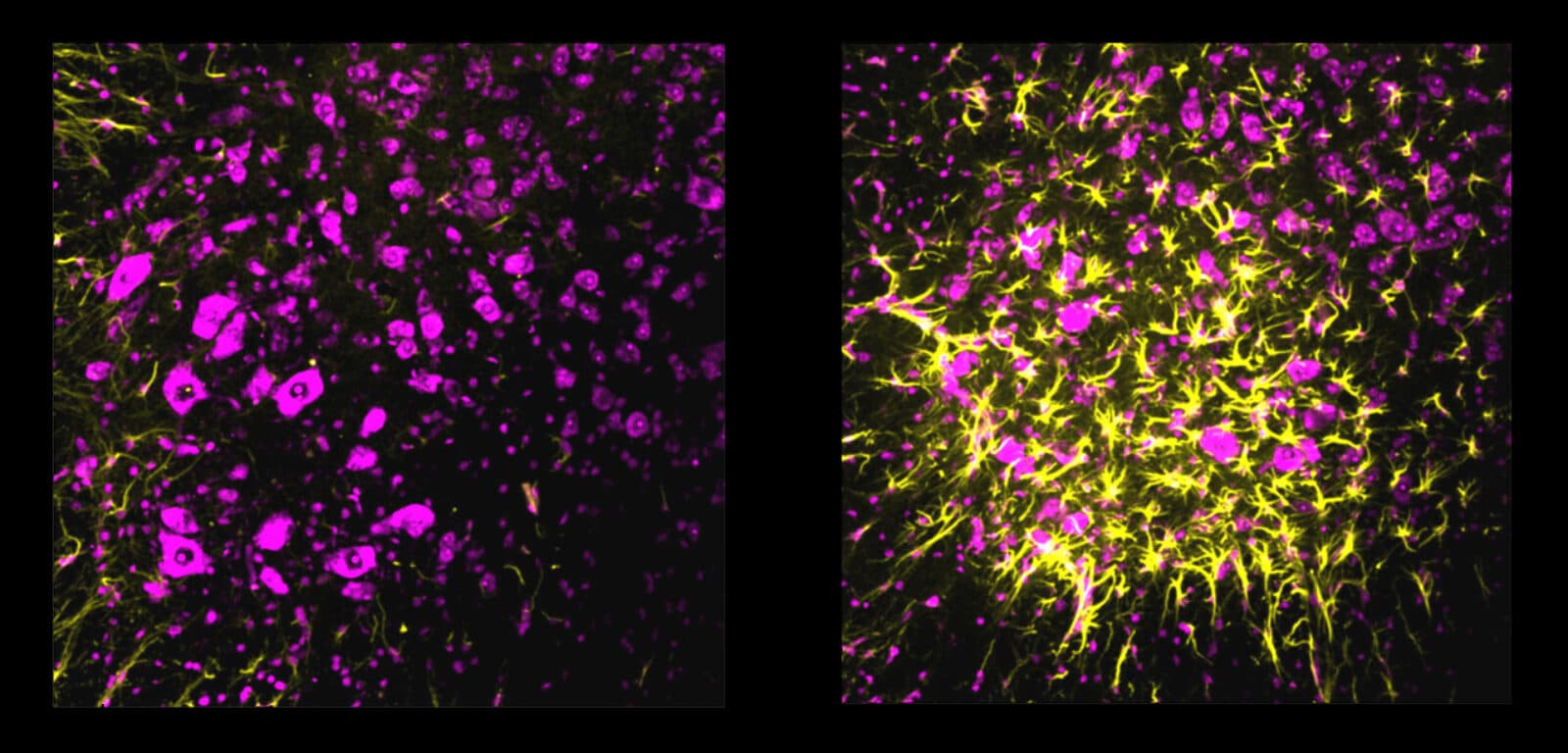
The Author
The Researcher
Homeostatic plasticity is a mechanism of neuronal resilience, which essentially describes how individual nerve cells respond to disruptions and keep our brains functioning normally throughout our lives.
Graeme Davis, the Morris Hertzstein Distinguished Professor of Medicine within the Department of Biochemistry and Biophysics, as well as codirector of the Kavli Institute for Fundamental Neuroscience, at the University of California, San Francisco, has studied the brain’s endogenous self-correcting mechanisms for more than 30 years.

Now he’s envisioning ways to boost these built-in self-corrective powers within our brains to better resist neurodegenerative diseases such as Alzheimer’s, amyotrophic lateral sclerosis (ALS), and dementia.
Many neurodegenerative diseases have a silent phase, where ongoing degeneration can be observed in some cases or it’s expected to be there but symptoms don’t show up. “In that silent phase where synapses are being nibbled away at, we believe these corrective mechanisms are being deployed to hold off disease, often for many years,” says Davis.
Promoting these endogenous self-correcting mechanisms may substantially prolong that window, he adds, and even help resist and delay cognitive decline after disease has kicked in.
“Perturbations come in the form of genetic disruptions, infections, injuries, or other kinds of things that affect cell function, and cells can detect the problem and resist it, recapitulate their normal activity levels and function—despite an ongoing perturbation—to keep brain function normal,” says Davis. “It’s a very powerful way to keep things working correctly.”
Back when Davis was first starting out in neuroscience, no one was thinking about these self-corrective mechanisms. He originally set out to change the way neurons connected with their target genetically, and manipulated molecules in an attempt to get neurons to “hyperconnect,” or connect less well to see what would happen.
“A surprise occurred early on,” he explains, “because although the cells became hyperconnected, there was no functional change in the way they communicated with each other. This was confounding for a little while, until we realized that it wasn’t that nothing had happened but rather a corrective response was engaged.”
The real excitement came once they also realized “wow, this is actually very generalizable and what we find in a fruit fly turns out to be exactly what’s going on in a mouse. And the same processes have been identified in humans,” Davis says.
His lab now works to perturb the nervous system to see how it responds. “We do careful perturbations to either change who cells contact, or hit them with toxins to carefully poison different aspects of the cell to see how they respond, and then quantify that to try to figure out which genes, molecules, and mechanisms can do these things,” Davis says.
One of the most remarkable aspects of this work, he emphasizes, is how accurate the whole system is. These endogenous self-correcting mechanisms have a corrective power of about 500%, which is truly enormous, and we carry this resilience around with us.
Davis likens it to the immune system, which is dormant until a pathogen shows up. “In many respects, that’s what these resilience pathways are doing,” he says. “When there’s a disruption, they kick in and correct it with remarkable accuracy because there’s so much precision in the way the brain has to work. It’s really remarkable how accurate these kinds of corrective mechanisms can be.”
His lab is also exploring diseases, which are a form of perturbation. “One disease we explored is ALS/Lou Gehrig’s disease in mice,” Davis says. “ALS is a rapidly debilitating disease that attacks your neuromuscular system, so we asked: What happens to these corrective mechanisms during that disease process?”
They recently wrote a paper describing how to stop the corrective mechanisms from working in ALS, and what happens if you do this.
“We now know enough of the molecules and genes involved to stop the resilience processes from working well,” Davis explains. “If we stop it in a normal animal, the animal is fine. It’s sort of an analogy with the immune system and pathogens: If there’s no problem and the nervous system is healthy, corrective mechanisms don’t turn on.”
But they discovered if you prevent corrective mechanisms from kicking in within the context of disease, the ALS models progress twice as fast. “Disease onset happens twice as early, neurons degenerate twice as fast, cells die twice as fast,” Davis notes. “It’s quite amazing, I don’t know of anything else as profound in that way.”
This suggested to Davis and colleagues that resilience mechanisms are powerfully keeping the early phases and maybe mid-phases of diseases in check. “We imagine we might be able to do something about this,” he says.
Eventually, our homeostatic systems get overwhelmed by disease and allow it to progress. “It’s not a cure, but we imagine it might be possible—given we know more and more about how these systems work—to potentiate, make everyone’s self-corrective powers as good as possible and maximize how everyone is able to resist these diseases,” Davis says.
The underlying cause of most neurodegenerative and psychiatric diseases remain unknown, which makes it difficult to know how to treat or create therapies for them. But the beautiful part here is that the self-corrective mechanisms we’re endowed with are “agnostic,” independent of cause.
If our self-corrective powers could be given a boost, it would be broadly applicable. You wouldn’t need to know whether you have a disease caused by gene A or gene B, or some interaction between a gene and environment—it would be equally appropriate.
“The breadth of applicability is exciting,” he says. “Catching cells and improving their function while they’re still in an almost normal mode—not catching cells on the verge of death and keeping them alive—allows them to resist the problem so they maintain their normal function.”
As far as a timeline to get there, “it’s a moment in science when all of a sudden the ball starts rolling downhill and it’s difficult to predict when therapeutic breakthroughs will happen,” Davis says. “But the ball is rolling and we’re moving in the right direction—rather than just exploring the possibilities.”
It’s time to start “thinking about how to capitalize on this for the benefit of human health,” he adds. “As a population of people, perhaps not all of us are equal in our abilities to mount these self-corrective resilience responses, so the goal is to bring everyone up to a maximal resilience.”