The Brain Activity Map Project
In 2011, neuroscientists and nanoscientists had an idea for revolutionizing our understanding the brain. Now that idea is a national challenge, as President Obama's BRAIN Initiative seeks to decipher the neural code that gives rise to our perceptions and experience.
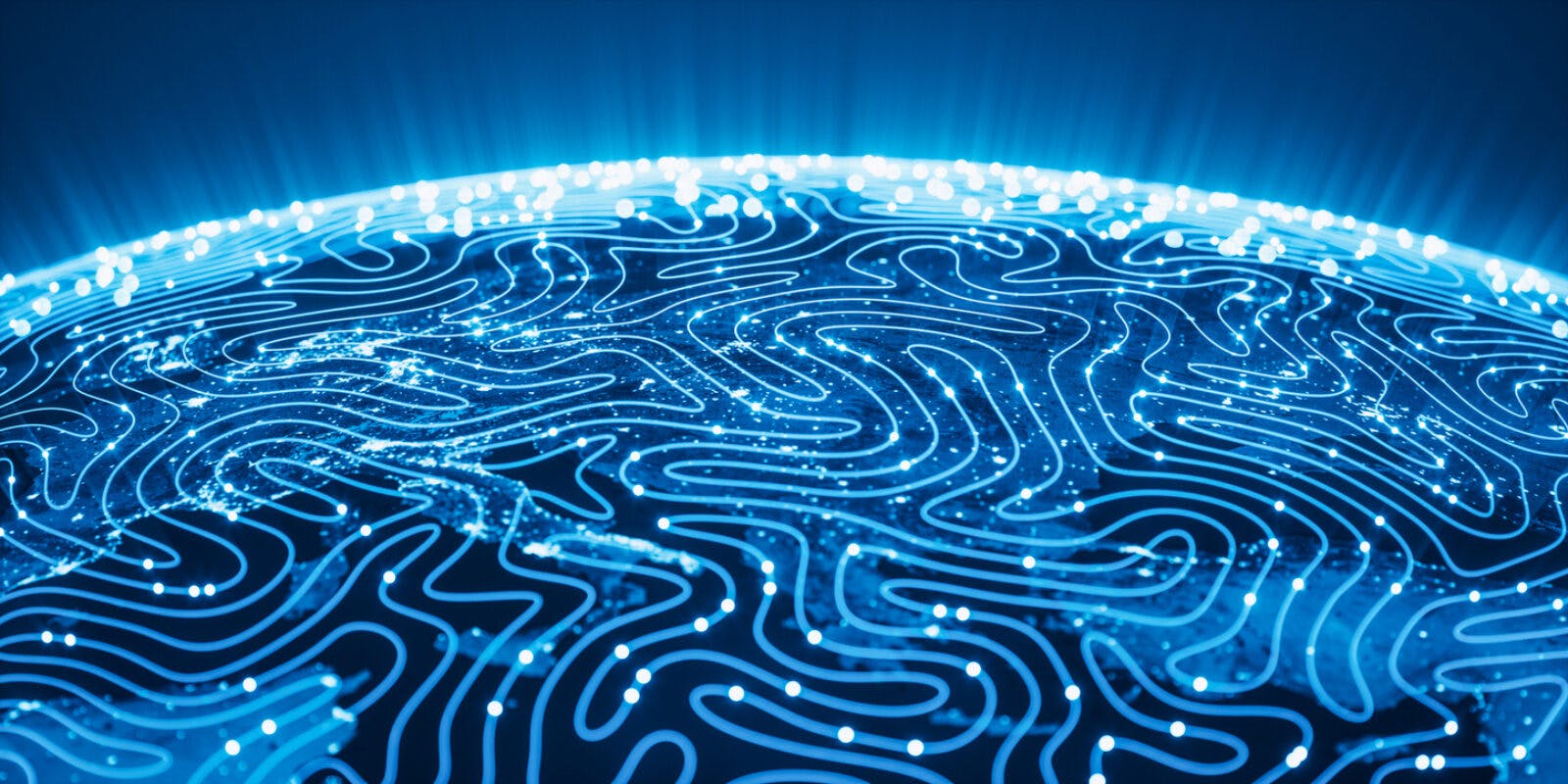
Despite more than a century of study, neuroscientists lack a fundamental understanding of how the brain gives rise to the complex thoughts, emotions and behaviors that make us human. Such knowledge would be invaluable for developing important clinical innovations to help diagnose and treat brain disorders as wide ranging as depression, stroke and paralysis.
86 Billion Neurons
The brain contains about 90 billion neurons – almost as many as the number of stars in the Milky Way galaxy. Communication between these cells is thought to underlie brain function, but studying that communication has proved enormously challenging. That’s partly because neurons form an almost unfathomable number of connections – somewhere in the range of 100 trillion, researchers estimate. Another difficulty is that these connections are constantly in flux, forming networks of neurons that consist of millions of interactions, continually shifting their shape and evolving based on what a person experiences. Current techniques allow neuroscientists to capture live signals either from just a few hundred neurons at a time or from large swathes of brain topography at once, but neither scale is sufficient to yield a comprehensive picture of brain activity. Instead, researchers believe that the activity generated by large assemblies of thousands or millions of neurons holds the key to understanding how the brain’s physiology gives rise to cognition and consciousness. Charting the activity of entire networks of neurons “is the major thing to be done to make a breakthrough in our understanding of how the brain works,” says John Donoghue, Director of the Institute for Brain Science at Brown University and a neuroscientist who studies how the brain encodes information and develops prosthetic devices for patients with paralysis.
The BAM project was conceived to do just that. The proponents of the BAM Project proposal focused on mapping large populations of neurons that would provide scientists an unprecedented understanding of the dynamics underlying this crucial middle scale at which consciousness and cognition emerge, but have so far lacked the tools to study. “For the first time, we would be getting close to the point where we can actually understand not just the way that the brain processes information, but how we hope and desire and think, our emotions and our goals,” says Terrence Sejnowski, a computational neuroscientist at the Salk Institute for Biological Studies in LaJolla, California and member of the Kavli Institute for Brain and Mind at UC, San Diego.
By focusing on the middle scale, researchers would finally be able to test hypotheses about the physiological basis of mind. For example, scientists could confirm or disprove whether an experience was truly linked with specific brain circuitry by first identifying networks activated when a rat encounters, say, an anxiety-provoking event, and afterwards stimulating that same network directly to determine whether the animal shows evidence of experiencing the same mental state. The proponents also saw BAM as dovetailing with a handful of other major initiatives recently launched to shed light on the brain. The Human Connectome Project, for example, has begun to map the pathways of neurons in the human brain – that is, the bundles of fibers that serve as the roadways along which electrical and chemical activity travels. In combination, the two mapping efforts would provide both the anatomical and the functional dimensions of the brain.
The BAM Project proposal, and now the BRAIN Initiative, share close parallels with another biology-based “Big Science” project – the Human Genome Project (HGP), a 12-year, $3 billion race to sequence the complete human genome completed a decade ago. When the HGP first appeared on the horizon in the 1980s, geneticists were extremely interested in sequencing but were not making use of advances in computing that could revolutionize their tools. “The field was over-ripe, but not moving as fast as it could,” says George Church, a geneticist and synthetic biologist at Harvard University and the Massachusetts Institute of Technology who played a leading role in the HGP. “The same thing is going on here. The neuroscience world has been ignoring the opportunities provided by recent advances in nanotechnology and synthetic biology.” Just as the HGP fueled a transformation in genomics both by changing how we think about medicine and by launching entire new areas of industry, so an iniative of this scale would have the potential to propel our ability to understand the brain to a new level and to create commercial opportunities that can provide an economic boost both in the US and abroad.
Technology at the Crossroads
Mapping activity in the brain will require several lines of technological development aimed at inventing the next generation of tools for measuring and instigating brain cell activity. Historically, researchers have recorded neuronal activity by using microelectrodes that physically pierce the brain. These probes, which are already being used in humans, are capable of both sampling neurons’ electrical properties and delivering voltage with great temporal and spatial precision. But the number of neurons at a time with which microelectrode arrays can interface must be increased several-fold while making sure the scale-up does not cause damage to brain tissue. That requires miniaturizing the technology as well as developing wireless devices that can transmit data into and out of the brain speedily and over long periods of time.
Another avenue of advancement involves the use of light-based technologies that can both detect and manipulate how and when neurons fire. Current microscopes and objectives are designed to focus light with great precision onto a single point in space, but targeting the activity of entire circuits of neurons will require the ability to image them in three dimensions. In parallel, researchers will need to devise molecular sensors that that can reliably register changes in electrical or chemical activity, as well as drive such changes, with single-neuron precision and in real time.
Scientists are also beginning to come up with completely novel kinds of molecular machines that consist of living cells biologically engineered to perform a specific purpose. Such tiny engines could be designed to gauge voltage changes in neurons and could deployed directly into the brain to perform the job. This armory of tools could likely spawn devices with medical and technological implications that cannot yet be predicted.
As these different techniques become available, researchers could simultaneously apply them to different model organisms. For example, animals such as worms or flies have simple enough nervous systems that they could be mapped in their entirety relatively quickly, to form testable hypotheses about complete cortical circuits. Studies in organisms such as mice, meanwhile, can be used to examine more complex functions or perfect novel approaches for use in humans. Meanwhile, human studies are already beginning to probe the relationship between disturbances in brain networks and disability and disease.
In order to make sense of this data, researchers will need computational resources for assembling, analyzing and sharing the masses of data that different components of the initiative will produce. That will involve harnessing approaches used in industries such as search engine technology, telecommunications and film animation, which are already adept at dealing with high volumes of information. So far, efforts to create comprehensive theories about how brain activity generates the mind have been hampered simply by the lack of data on how large assemblies of neurons function. This initiative however would provide the foundation for researchers to begin developing truly testable hypotheses of brain function. “The reality is, until you’ve collected the information, you can’t really make good models of how things work,” says nanomaterials scientist Paul Alivisatos, a nanomaterials scientist at the University of California, Berkeley, who specializes in developing nanoscale technologies.
Into the Future
Many laboratories are already working on developing the technologies that an initiative like this will require. Because of its scope, however, success will require a centralized, collective effort on a grand scale. In turn, it will generate multiple lasting benefits.
First, it will produce a slew of novel techniques that will filter into neuroscience labs around the world, and allow researchers to make discoveries that are not possible today. “Once we start to correlate particular patterns of activity to behaviors or mental states,” says Rafael Yuste, a neuroscientist at Columbia University who studies mammalian brain circuitry, “we will reinvent neuroscience as a field.” The initiative will also re-chart the landscape of neurological and psychiatric disorders. A thorough understanding of the underlying chemistry and electrical activity of the brain will lead to a new suite of approaches for precise diagnosis and effective treatments that allow clinicians to restore disrupted or damaged neuronal circuits.
The collaborative roots in nanotechnology and engineering of a project such as this will also have far-reaching effects. Smart miniature sensors and other tools developed for the purposes of measuring brain activity will be based on technological advancements with applications in many other fields. And just as the HGP generated as much as a 200-fold return on the $3.8 billion dollars invested in the project, new industries launched by innovations made within the BRAIN initiative are likely to bring a similar level of economic benefit. Finally, the intensive focus on cross-disciplinary research has the potential of reaping the rewards of a different sort of investment as well – one in training a new generation of scientists who will seek to continue working at the intersection of biological and physical sciences research.

Disorders of the Brain and Mind
Disorders of the brain and mind – schizophrenia, Alzheimer’s disease, depression, and epilepsy, to name just a few – take an enormous toll on individuals, their families and society at large. Researchers increasingly believe that many such conditions are closely associated with disruptions in brain activity patterns. Mapping these patterns will thus empower researchers to develop tools for rebalancing circuitry when it goes awry.
Already, researchers have developed devices such as deep brain stimulators for treating Parkinson’s disease, cochlear implants for restoring minimal hearing in profoundly deaf people, and a computer interface called BrainGate that allows fully paralyzed individuals to accomplish simple tasks via a robotic arm. These interventions are beginning to make use of our rudimentary understanding of brain circuitry, but understanding the language of the brain with a high level of precision will sharply boost researchers’ capabilities to recreate some of its more complex functions. Knowing how specific patterns of activity are switched on and off might suggest ways that educators could engage certain networks to facilitate different types of learning, for example, or could serve as a blueprint for developing a new wave of artificial intelligence devices.

Re-Imaging Neuroscience
Today, most people with a basic science education know what a neuron is. But it was only in the late 19th century that Spanish neuroanatomist Santiago Ramón y Cajal convincingly showed that such cells – rather than an interconnected net of tissue – formed the basis of the nervous system. He used an assortment of anatomical tracing techniques to label neurons in their entirety – a cell body with a long axon extending out in one direction and the branched tendrils of dendrites protruding from the other. In the ensuing decades, researchers explored the electrical and chemical properties that make neurons tick. Australian neurophysiologist John Eccles and his colleagues in 1951 first managed to insert miniscule-tipped probes called microelectrodes directly into a neuron from the central nervous system to measure its responses to signals from neighboring neurons. The following year, based on experiments in the giant squid axon, British biophysicists Alan Hodgkin and Andrew Huxley described how ion flow across the cell membrane generates the electrical spikes – called action potentials – that constitute these signals. Based on those discoveries, researchers turned their attention to investigating the environmental cues or behaviors that make neurons fire. Two neuroscientists working in the U.S. in the 1960s and 1970s, David Hubel and Torsten Wiesel, discovered that in the visual system, different kinds stimuli – a moving line, for example, or a particular color – would turn on certain neurons and not others. Since then, scientists have developed ever-more-sophisticated methods for studying these sensitivities, which are called receptive fields, in individual neurons.
The focus on properties of single neurons was a driving force of neuroscience in the 20th century and remains so even today. But it has proved to be a limited approach. Just as you cannot hear a symphony when only the first violinist plays alone, so the complexities of the brain likely cannot be grasped by examining the functional properties of each individual neuron, measured alone.
More recently, neuroscientists have been able to take a wider view of brain function using new technology to track activity levels across the entire brain instead of individual cells. An approach called functional magnetic resonance imaging (fMRI), for example, records changes in blood flow that occur when a spark of activity passes through a particular brain region. Another technique dubbed magnetoencephalography (MEG) maps magnetic fields in the brain produced by electrical signals; a similar approach, transcranial magnetic stimulation (TMS), selectively stimulates parts of the brain and is already being used to treat severe depression, migraine headaches and other conditions. These newer methods, however, can’t capture the activity of individual neurons, and the picture they provide is therefore too blurry for scientists to directly connect changes in function to distinct thoughts, behaviors, or states of mind. Most importantly, these methods do not reveal the mechanisms by which these elements of cognition occur.