Breakthroughs in Reading Molecules
by Alan S. Brown
Research highlights from Kavli Institutes in Nanoscience
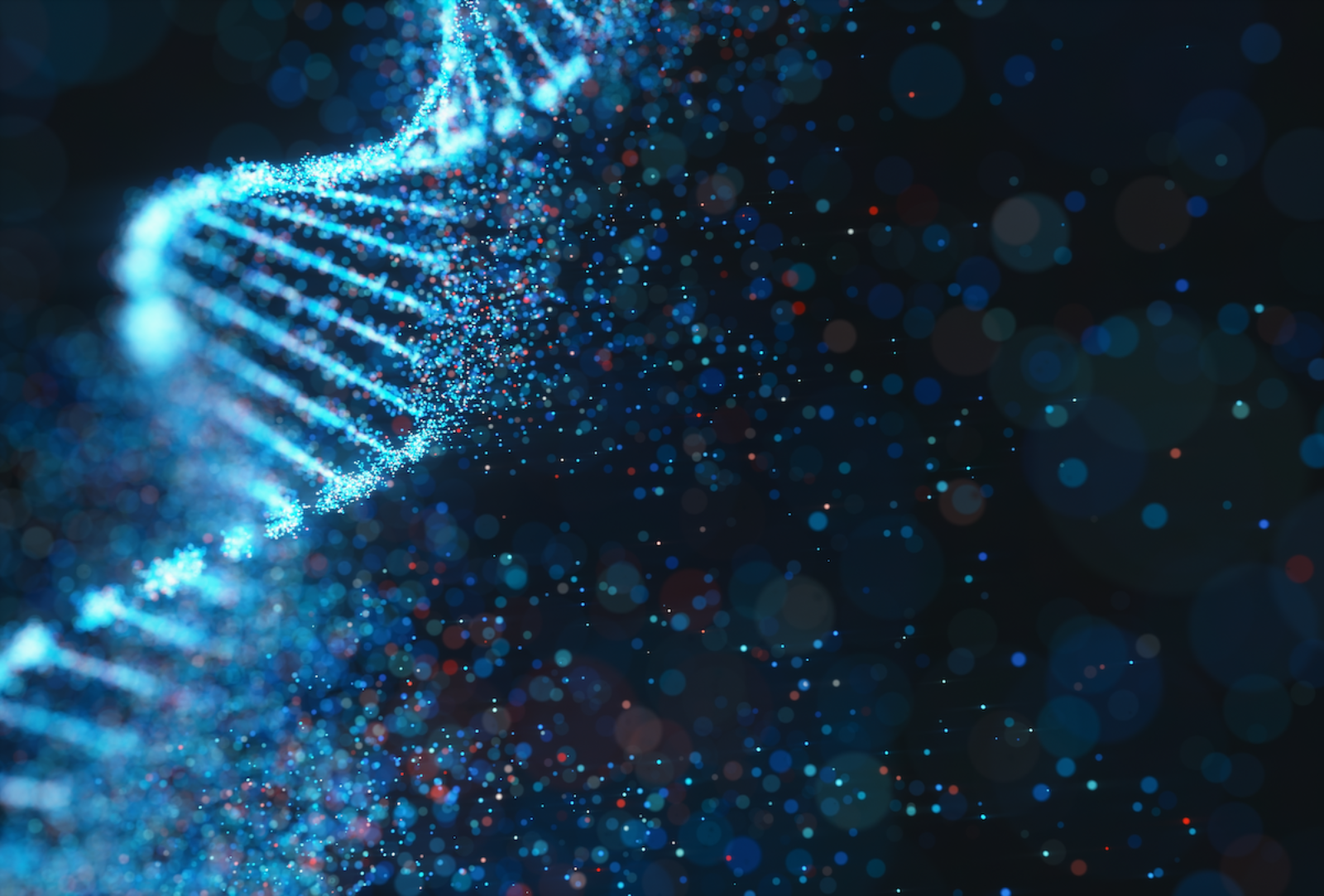
The Author
How do you know what a protein is really made of? The answer lies in a cool nanoscience technique called DNA sequencing. This involves pulling a strand of DNA (or RNA) through a nanoscale pore. As each of its four amino acids pass through, it produces a unique electrical current that identifies it. Proteins, however, are much more complex than DNA. They consist of 20 types of amino acids and their composition may change once created. Yet Kavli Institute researchers have found a way to read them. Meanwhile, other Kavli-affiliated researchers have combined databases about RNA in damaged muscle cells to identify a remarkable 365,000 different cell types involved in healing. Both techniques could open a door to new types of medicines. Also this month, we examine a way to use the flaws in 3D printing to make a better metal part, advances in materials that can pull water from desert air, and a report about recycling potentially tens of millions of lithium-ion batteries.
Revealing the truth about single proteins. Proteins are shapeshifters. Because cells make them from a DNA blueprint, we can predict which amino acids are in them. But once made, proteins do not always reflect their original DNA instructions. Rather, they may change like a house renovated several times over the years. This is why identifying proteins in a cell requires analyzing many, many samples, yet often misses proteins that are too rare to find in volume. The solution may be a technique developed by a team led by Cees Dekker of the Kavli Institute of Nanoscience at Delft Technical University. Using DNA sequencing, the researchers found a way to untangle the convoluted twists, turns, crossovers, and knots of a typical protein and run it through a nanopore reader. The technique could one day to identify rare proteins that might be involved in disease.
The overwhelming complexity of muscle repair. From black-and-blue bruises to punctures and tears, muscle injuries set off a torrent of reactions in the body. Stem cells begin splitting and differentiating into thousands of specialized cells to repair the damage. Many of these repair cells last only a few hours or even minutes, making their functions—or even identity—hard to pin down. Now, Iwijn De Vlaminck, a member of the Kavli Institute at Cornell for Nanoscale Science, and his colleague Ben Cosgrove, have found developed a database that has identified 365,000 cell types involved in muscle repair, including hundreds and hundreds of transient stem cell offspring. The database grew out of their work to sequence the RNA of individual cells, which identified 35,000 cells. Knowing that other researchers had done similar work, they decided to combine everyone’s data—88 databases—into a single format scMuscle. The new database provides a more complete (if not yet clearer) picture of cells involved in healing and their proximity to one another, and could be used to develop advanced diagnostics for some muscle diseases.
Reimagining machines. Try to imagine, for a second, a robot hundreds of times smaller than the millimeter markings on a ruler, or a nanomachine hundreds of times smaller than that. What would they look like? Ordinarily, we envision robots with gears, levers, wheels, and other moving parts. But that is not how Itai Cohen, a member of the Kavli Institute at Cornell, imagines them. At the microscale and nanoscale, molecular forces might keep those mechanical devices from working the same way they do in larger devices. In the past, Cohen has sought to create tiny robots with moving parts that he can assemble using origami-like folds. Now, working with post-doc student Italy Griniasty and Cyrus Mostajeran of University of Cambridge, he goes a step further: he is building robots from a single sheet of liquid crystal elastomer that can morph from shape to shape . By controlling the alignment of the molecules in the sheet and the type of stimuli to which they respond, he believes he has found a way to create complex motion in microscale objects. The work is still theoretical, but one simulation shows a disk-like robot that swims through viscous materials by alternating between conical and flat shapes.
Pulling water from desert air. Several years ago, Omar Yaghi, co-director of the Kavli Energy NanoScience Institute at University of California, Berkeley, discovered a molecule that could pull water from desert air. Now, the race is on to make it efficient enough to use in the real world. Recently, Yaghi collaborated with scientists at University of Chicago and other research centers to achieve a better understanding of its chemistry. The molecule in question is called a metal-organic framework (MOF), which is made of linkers and rods and is exceptionally porous. As air flows through it, electrical charges in the molecule trap the water while letting air pass through. The researchers found that the MOF pores each do this by bonding the first three water molecules to the linkers and rods. The other water molecules then bond to the water molecules. Now that they understand the mechanism, the team plans to use machine learning to develop MOFs that can hold more water.
Can we recycle our electric future? In 2019, the world produced more than 90 million vehicles. Assuming we make as many electric vehicles in the future, the world will have to find a way to eventually reuse or recycle tens of millions of lithium-ion batteries ever year. A team led by Lyndon Archer, a member of the Kavli Institute at Cornell, and his colleague, Fengqi You, have completed a life-cycle analysis that makes some interesting points. Take, for example, cobalt. This metal is expensive, energy-intensive, and associated with child labor, yet it also suppresses component oxidation and enables battery recycling. Any replacement will have to provide similar functionality. They also made a case for reusing lithium-ion batteries to store solar and wind power for use at night or when there is no wind. This would reduce the battery’s overall carbon footprint by up to 17 percent. The study also called for government incentives to recycle batteries after they have been reused.