Solar Power: Is It Time for the Big Push?
by Alan S. Brown
In the coming years, the fastest growing source of renewable power may be solar energy. But first, it must overcome some technological challenges.
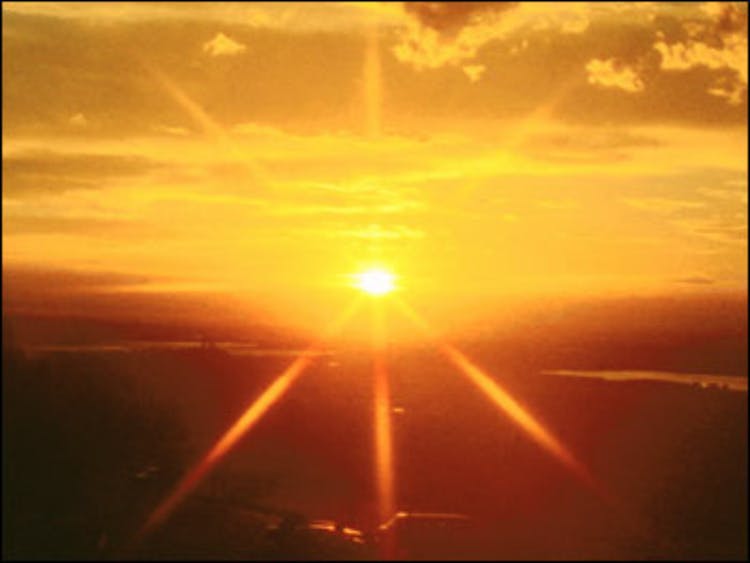
The Author
The Researchers
For conventional photovoltaics, which convert sunlight to electricity, challenges remain. Commercial panels are rarely more than 20 percent efficient and while some experimental systems achieve 44 percent efficiency, they are not economical enough to manufacture reliably. Moreover, to reach solar's true potential, ways are needed to store solar energy for use at night.
Recently, three experts, moderated by Harry Atwater, discussed how to move solar technology from the lab to commercial reality.
- Harry A. Atwater, Jr. – Director of the DOE Energy Frontier Research Center on Light-Material Interactions in Solar Energy Conversion. He also heads the Resnick Institute for Science, Energy and Sustainability, California Institute of Technology's largest endowed energy research program, and is a member of Caltech’s Kavli Nanoscience Institute (KNI).
- Nathan S. Lewis – Principal investigator of the Joint Center for Artificial Photosynthesis, the U.S. Department of Energy’s Energy Innovation Hub in Fuels from Sunlight at Caltech, and member of KNI. He is a pioneer in artificial photosynthesis, studying how light causes electron transfer reactions as well as novel uses of conducting organic materials and composites.
- Albert Polman – Director of the Dutch Foundation for Fundamental Research on Matter's Institute for Atomic and Molecular Physics in Amsterdam, The Netherlands. He is a pioneer in applying nanophotonics, the control of light at the nanoscale, to improve the efficiency of solar cells.
- Michael Wasielewski – Clare Hamilton Hall Professor of Chemistry at Northwestern University, and director of the Argonne-Northwestern Solar Energy Research Center. His research focuses on light-driven charge transfer and transport in molecules and materials, natural and artificial photosynthesis, and nanoscale materials for solar energy conversion.
The following is an edited conversation led and moderated by Dr. Atwater.
HARRY ATWATER: Let's start with photosynthesis. It is not about generating electricity, but about producing fuel that we can store and use later, right?
NATHAN LEWIS: Yes. There is a gap as big as the Grand Canyon in the low-carbon power environment: How do you store and transport energy for applications that can't be electrified, like aircraft and ships?
We can make electricity from solar power, then convert it into hydrogen fuel by splitting water, but that makes no sense. It's not very efficient. It would make better sense to make the fuel up front, with a specialized photosynthetic solar system, and get your electrical product from another set of photovoltaics.
ATWATER: Photosynthesis is an extremely robust process that plants have used for billions of years. But can we make it efficient enough for commercial fuel production?
MICHAEL WASIELEWSKI: Photosynthesis provides a good working model for solar energy conversion. We've all heard it is only 1 to 2 percent efficient at converting sunlight to sugars, which plants use as fuel. But those calculations are based on the overall process, both light capture and a long series of biochemical reactions to make the sugars. And if you look at the business end of photosynthesis, light capture is nearly 100 percent efficient, and its follow-up conversion to stored charges approaches 40 percent. The initially stored charges provide the energy to drive the subsequent biochemistry to make sugars.
Now it is true that photosynthesis uses proteins and other organic molecules that are not sturdy, but they provide good working models for how we can convert and store solar energy. We can really think of research in photosynthesis as biomimetic or bioinspired. We've done a lot of work on the biomimetic side, where we try to use some of the natural components of photosynthesis to assemble solar energy conversion systems. But as we learn more, we are inspired to ask, “What can we learn from this process that we can apply to more robust materials?” Recent research focuses on using this “bioinspiration” to design and build systems that work like natural photosynthesis, but are made of far more robust materials.
LEWIS: I agree, and I'd like to make a few points. I like to think of solar energy as an inevitable technology. After all, electric motors are 85 percent efficient and combustion engines are at best 30 percent efficient, so we are going to electrify wherever we can.
If we look at the overall energy efficiency of photosynthesis, it's only 1 or 2 percent. But that's not how we should think about it. Just because birds have feathers doesn't mean that airplanes should. Airplanes are bigger and faster than birds. We want to apply physics, chemistry, engineering, and nanoscience, and achieve the same degree of improvements with solar energy.
ATWATER: Lately, we've seen remarkable improvements in the efficiency of photovoltaic cells, which turn sunlight into electricity. Are we getting close to broad commercialization?

ALBERT POLMAN: Today you can buy 20 percent efficient solar panels and it's not a stretch to imagine that will rise to 30 percent. If you wanted to put all those cells in one spot to supply all the world's power needs, it would take up quite a large area. But in the real world, we would distribute them all over the globe -- some on the roofs of homes, schools, and office buildings; others in solar farms. We are getting to the point where we can do something like this at a very large scale.
ATWATER: Photovoltaic cells also face an energy storage problem. Hawaii, for example, already generates about 30 percent of its power from solar, and cannot generate more because it has to run central oil-fired power plants for electricity at night. What do we do?
LEWIS: This is a big problem. In the 2009 report, America's Energy Future, the National Academy of Science projected that we could get 10 percent of our total electrical energy from non-hydropowered renewables by 2020 and 20 percent by 2030. But to get above 20 percent, we will need to develop entirely new ways to store and distribute electricity. To do that, we need to pursue two approaches. First, we need to boost efficiency so we get more power for a given area. Second, we need to really scale up manufacturing to make this technology cheap. Right now, making solar cells is like making other highly engineered technologies, such as silicon chips. What we need are technologies that let us churn it out inexpensively, like newspaper or bubble wrap.
ATWATER: When solar cells were originally conceived, they didn't incorporate what we would call nanotechnology. What can nanotechnology do for photovoltaic and photosynthetic systems?

WASIELEWSKI: One area where nanotechnology has made great strides is the use of self-assembling molecules and materials to build efficient structures for high-volume, low-cost production. Let me give you an example. Having highly ordered materials in photovoltaic cells improves performance. Yet many cutting edge nanophotonic materials are poorly ordered. It is well known that flat molecules will stack in specific ways, similar to the way north and south poles on magnets will cause them to attract and repel in certain ways. We are developing ways to make those flat nanophotonic molecules align like coins in a stack. By taking advantage of these nanoscale molecular properties, we hope to apply self-assembling molecules to a substrate in ways that they form ordered structures for photovoltaic cells without expensive processing.
ATWATER: Mike [Wasielewski] talked about using nanotechnology to build structures from the bottom up, starting with individual molecules. Albert, what about working from the top down?
POLMAN: One of my interests is soft printing, using something like a rubber stamp to print a solar cell’s nanoscale patterns on a plastic substrate. In the last couple of years, several companies have come with processes that print nanostructures on 1-meter-wide sheets of plastics, similar to how we print newspapers.
I did some quick calculations with my class recently. We asked what it would take to print enough solar cells to cover the total energy needs of the earth in 10 years. You could do it with just one factory if you could print solar panels as fast as we print newspapers.

ATWATER: We've talked about using everything from conventional semiconductors to organic molecules for solar technologies. Do we have all the materials we need for photovoltaic and artificial solar fuel cells?
WASIELEWSKI: We don't. All the avenues have certainly not been explored. This is especially true of hybrid materials, combinations of inorganic and organic materials. This is, in part, a function of how we do research. In the past, the materials community was more segmented in terms of its research interests, so one researcher would specialize in inorganic materials and another in organics. We're just beginning to get out of those niches and explore a wider variety of hybrid materials. We need to learn how to do this rapidly and not get trapped in intellectual cul-de-sacs.
ATWATER: The rule of thumb is that it takes 15 years to develop materials the old way. How do we accelerate the rate of progress?
WASIELEWSKI: Right. We need to engage with manufacturers and end-users of the technology as soon as possible, rather than spend years doing lab demonstrations before anyone talks with industry. We need to take advantage of manufacturers' expertise in how things are really done. On our part, we need to let them know about promising materials sooner, so they start to think about commercialization pathways earlier in the process.
ATWATER: Are there other things we can do to speed progress?
LEWIS: There is some clear consensus on critical needs. For example, we need inexpensive, abundant catalysts to split water into oxygen and hydrogen, which we can store and use for fuel. When we split water, we currently catalyze the reaction with iridium or platinum, but these elements are too scarce and expensive. Nature knows how to do this, fixing water and carbon dioxide into sugars for fuel. Manganese gets us part way there, but we still have a long way to go.

LEWIS: Another thing we can do is to embrace combinatorial research. This is not going to replace directed scientific research, but it does give us a way to rapidly create and screen lots of chemical compounds for photovoltaic activity.
ATWATER: How does it work?
LEWIS: We prepare batches of mixed compounds by a variety of means, apply them to an array of LCD-derived pixels, light up the pixels, and measure the electrical current generated on each pixel. Instead of synthesizing one compound per month, we can use this method to test thousands of randomly synthesized compounds per day, then take a closer look at the best performers. We can also build an enormous database from the experiments, and try to validate structure-performance relationships in ways we never could do before. This could create a very powerful link between data and theory.
ATWATER: Albert, you're investigating nanoscale phenomena. Are there things we can do to move forward there?
POLMAN: Using nanostructured materials to make solar cells is only about 10 years old. We're interested in the nanoscale confinement of light, how we can catch light in more efficient ways to split water. I work more on the physics side, but there is a separation between what I do and the chemistry needed to make this work. To make things happen faster, we need to have the disciplines talk to one another more than in the past.
"[T]o commercialize solar technologies, we must get past the valley of death – that big gap between demonstrating a technology and finding someone to invest $100 million for large-scale manufacturing." – Harry A. Atwater, Jr.
ATWATER: I want to go back to something Mike said a few minutes ago, about making our research more sustainable to attract the best young researchers. Are there things you can do in your labs to make this happen?
WASIELEWSKI: One thing we do is set aside some resources to promote collaborative and cooperative research in a global setting. We find that when people working in our lab spend time in other labs, they get a better appreciation of the types of science and style of research that other workers do, and they can bring that back to us. The reverse is also true, so that this type of back and forth is essential to developing research rapport across disciplines, and we should try to find new ways to do more. We should make it part of the graduate student educational process, so we ultimately wind up with young people who feel that collaboration and cooperation is the normal way of doing business, instead of narrowly segmenting their research.
Everything takes resources, but this involves relatively modest costs. An additional add-on for graduate cooperative work would pay very great dividends in making sure everyone is taking advantage of all the different types of science in our community.

ATWATER: I agree, we need new modalities. At Caltech, for example, the Resnick Institute endows energy science fellowships for graduate students who develop proposals that have two or more faculty as advisors and mentors. The students get really synthetic ideas in ways that they wouldn’t get in working for a single professor alone. Now, I want to move onto questions about industry support. For several years, private investors made a significant commitment to photovoltaics, but the sharp decline in solar cell prices has disrupted many businesses and now they are leaving the field. How do we bounce back?
POLMAN: I'm in Europe and we're not having such a bad time with research funding, although budgets are declining right now. Among the political parties here, we see a lot of interest in energy research. When it comes to taking the next steps after research, this is a very difficult question to answer.
"I did some quick calculations with my class recently. We asked what it would take to print enough solar cells to cover the total energy needs of the earth in 10 years. You could do it with just one factory if you could print solar panels as fast as we print newspapers." – Albert Polman
WASIELEWSKI: That's why in the US our relationship with the corporate world has to become a lot better. The economy drives everything and politics go hand-in-hand with that. If we can convince industry this will be a profit center 20 or 30 years down the line, they will help us convince everyone who needs to be convinced. Right now in the United States, though, natural gas is the 800-pound gorilla in room because fracking has made it so available and cheap. But in terms of reducing carbon emissions, it gets us only half the distance. It is a near-term solution, but we need solar to move forward.
LEWIS: There are also energy gaps we have to bridge. We need to find a way to decarbonize the 40 percent of transportation that can't be plugged in, like ships, trucks, and airplanes. We could do that with hydrogen, but it will require energy storage.