Catalyst Chemistry Could Turn Emissions into Green Fuels
by Alan S. Brown
Using an electron microscope developed at the Kavli Institute at Cornell for Nanoscale Science, Yao Yang is helping elucidate copper catalysis at the Kavli Energy NanoScience Institute at UC Berkeley.
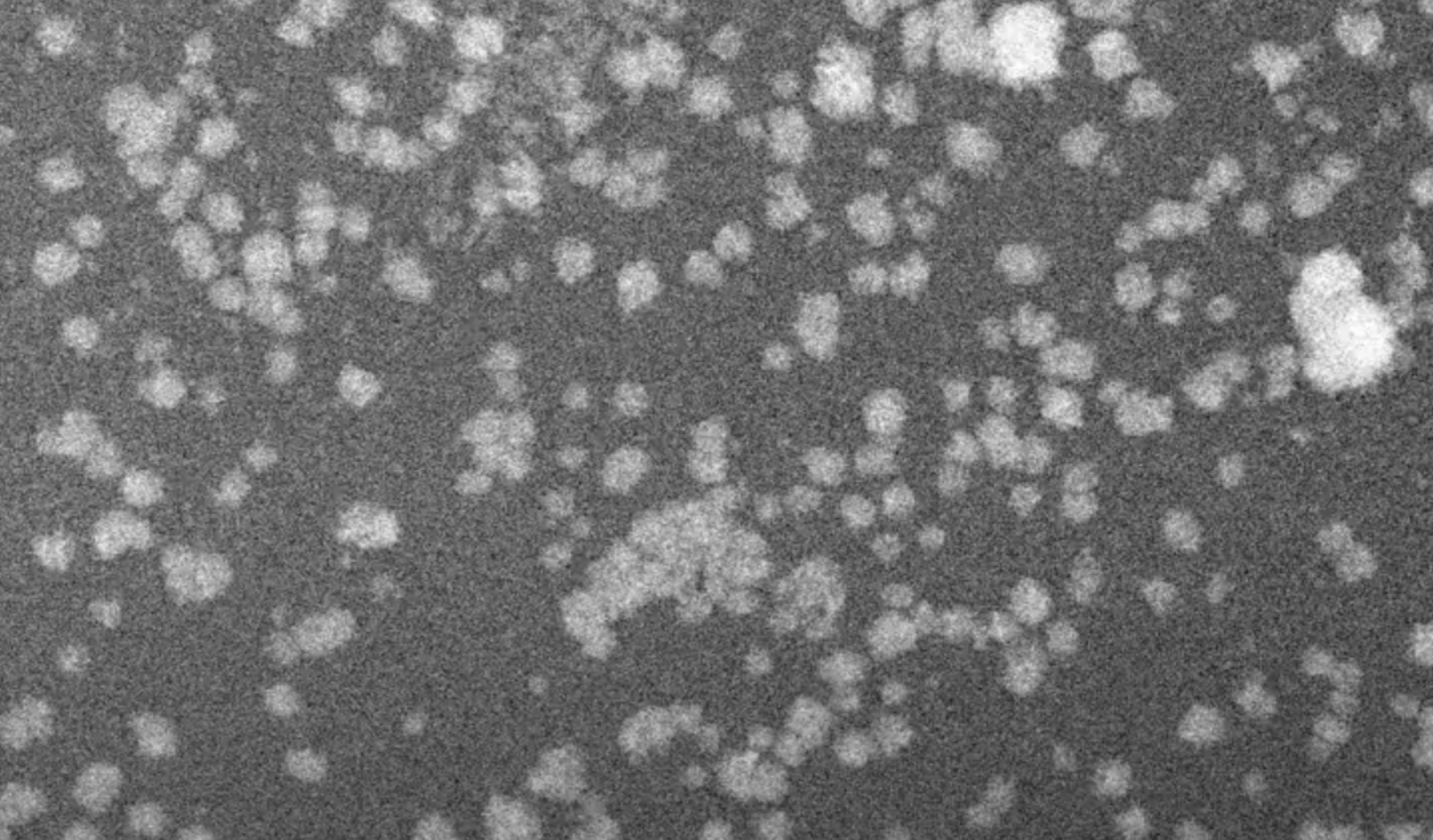
The Author
A new movie has drawn rave reviews, though mostly from chemists and physicists. It portrays the evolution of nanoparticles of copper, the only material known to efficiently transform carbon dioxide into multi-carbon molecules like those found in fuels, drugs, and other organic chemicals.
The video was produced by Yao Yang, a Miller postdoctoral fellow working with Peidong Yang, director of the Kavli Energy NanoScience Institute at UC Berkeley. Yao and his collaborators used an electron microscope developed by researchers at the Kavli Institute at Cornell for Nanoscale Science. The movie captured for the first time how small particles of copper cluster together to generate regions that react with carbon dioxide when activated by an electrical potential.
By better understanding this process, the researchers hope to develop copper catalysts with sufficiently high efficiencies to make it practical to recycle CO2 from automobiles and power plants back into fuels without adding any additional greenhouse gases to the atmosphere.
The Road to Physical Chemistry
Yao grew up in Henan province in central China. When he found himself torn while choosing his major as he entered college, his father offered him some advice, saying, “The world will always need chemists.”
“I didn’t really even know what chemistry was,” Yao said. Yet he quickly took to lab work, thanks in part to the hands-on skills he learned from his father. “I had no fear of handling any experiment,” he said. “That was helpful, because chemistry often starts with experimental exploration of unknown phenomena.”
As part of an investigation into alkaline fuel cells, he quickly realized that better catalysts held the keys to better fuel cell performance. “While most other things produced incremental advances, a better catalyst could improve performance by orders of magnitude,” he said.
Yao’s advisors at Wuhan University, Lin Zhuang and Li Xiao, encouraged him to apply to the Ph.D. program at Cornell University, as both Zhuang and Xiao had been visiting scholars in Héctor Abruña’s lab there. Once at Cornell, Yao worked with Abruña, who is a member of Cornell’s Kavli Institute. One of Abruña’s colleagues and close collaborators was David Muller, co-director of the Kavli Institute, who was revolutionizing the capabilities of electron microscopes.
Muller was developing a technique called electron ptychography, which looks at the patterns created by overlapping scans of an object to compute the shape of the objects (or atoms) that caused those patterns. Over the years, Muller’s lab has boosted the system’s speed, improved its abilities to scan thick samples, and pushed its resolution into the picometer (1,000th of a nanometer) range—small enough to see the spaces between individual atoms.
Yao earned Muller’s support to use scanning transmission electron microscopy (STEM) to perform atomic-scale characterization of precious-metal-free electrocatalysts used for alkaline fuel cells and electrolyzers. Yao and his collaborator Yu-Tsun Shao demonstrated the first example of four-dimensional STEM in liquid, a key technical breakthrough to enable this exciting story.
Yao’s work caught the attention of UC Berkeley’s Peidong Yang. For years, Professor Yang had been trying to develop systems that used sunlight and nanoscale catalysts to transform CO2 and water into long-chain carbon molecules. While copper nanocatalysts could achieve this, they produced a potpourri of reaction products. It was simply too expensive to separate the desirable chemicals from the others.
Peidong Yang wanted to form a small number of products (or even a single product) at a time. To do that, he needed to fully understand how the catalysts behaved under reaction conditions. Realizing that Yao might have the tools to answer those questions, he encouraged him to apply for a Miller fellowship and work with him at UC Berkeley.
Windows into the Nano World
“Peidong wanted to know what he had to control so that copper would make the chemicals he wanted,” Yao said. “What was the best shape: wires, cubes, atomically thin two-dimensional sheets? What about size? Did their morphology change when the reaction began? We had almost no insights into how they looked under reaction conditions. It was not until the past few years that we have had the tools to answer these questions.”
Finding an answer, however, presented a problem: The reaction that turns CO2 into long-chain molecules takes place in water, but electron microscopes operate in vacuum. “Unfortunately, vacuums and liquids almost never get along,” Yao said.
To bridge that gap, Yao needed to develop a small cell to hold the liquid inside the microscope’s vacuum. The easy part was the container, which would hold an electrode coated with pure copper nanoparticles, water, and sodium bicarbonate to convert CO2 into valuable products.
The hard part was the front and back windows on the container. On the one hand, they needed to be sufficiently strong to contain the aqueous mix, despite a seven-orders-of-magnitude difference in pressure. On the other, they had to be thin enough to allow electrons to penetrate and exit the cell.
Yao needed lots of electrochemical cells to create his movie. The first three-electrode electrochemical liquid-cell microchip was made possible thanks to a decade of prior work from researchers including Megan Holtz, Yingchao Yu, and Burak Ülgüt.
The resulting cell chips measured 1.0 centimeter by 0.5 centimeter and are handled under an optical microscope. Everything is done in a clean room because the cell’s windows are so thin, just 50 nanometers, that a collision with a speck of dust could crack them.
Closing in on Copper Catalysts
The lack of an appropriate cell was not the only reason researchers had never tried to take electron microscope images of liquids before. Another problem was that conventional equipment accelerated electrons to such high energies that the electrons would boil any liquid and dramatically affect the reaction rates inside the cell.
Muller’s electron ptychography system, employing an electron microscopy pixel array detector (EMPAD), was different. Its detector was so sensitive, it could produce a high-quality image using a several-orders-of-magnitude lower dose of electrons relative to a conventional system. This enabled it to scan copper’s interaction with CO2 without damaging the contents of the cell.
At least, that is what Yao thought. To confirm that this was in fact the case, he had to demonstrate that his chips exhibited the same chemical behavior produced by similar reactions on a chemist’s lab bench, except on a much smaller scale. It took hundreds and hundreds of reactions to demonstrate that the two were, indeed, equivalent.
This was not easy, since EMPAD’s low-intensity electron beam made it harder to separate the signals created by chemical reactions from the background noise created as electrons passed through the liquid sample. Ultimately, he compromised by lowering the resolution, so instead of viewing atoms, he looked at structures down to the order of one nanometer. Still, this was sufficient to probe the behavior of the copper catalysts.
“We were just thrilled by the possibility that one could watch real-time movies of chemical reactions at the nanometer scale,” said Yao. “We could watch chemistry in action!”
Yao planned to probe the liquid cell with the EMPAD and a technique from Berkeley’s Advanced Light Source called resonant soft X-ray scattering (RSoXS) developed by Cheng Wang. By using the same liquid cell, he could ensure that none of his measurements were caused by differences in the experiment’s environment. Using RSoXS, he could view millions of particles at a time, producing a big-picture view of catalyst behavior and chemical reactants and products.
To get closer, though, he needed the EMPAD. While others had observed copper electrocatalysts clumping together when an electrical current passed through them, that was all they could see. EMPAD’s single-electron sensitivity enabled Yao to image “electrochemical scrambling” (a term coined by Peidong Yang), where the oxide layer that prevented copper from reacting with CO2 disappeared on some parts of the agglomerate, generating regions of pure copper that catalyzed the reaction.
But not every exposed region of copper participated in the reaction. The EMPAD movies showed that some copper atoms were tightly bound to one another. In other areas of the agglomerate, however, copper atoms had many dangling bonds. These bonds captured passing CO2 and water molecules, converting them into multi-carbon chains. And they appeared only on nanoparticles that were seven nanometers in diameter or smaller.
“This was what we were missing before,” he said, “the details of what happened when the catalyst changed from starting particle to cluster. Those Cu nanograins essentially serve as tiny chemical factories for converting CO2 into liquid fuels.”
Now, for the first time, researchers have a handle on the chemistry driving copper’s remarkable catalytic properties. They understand how agglomeration works, what particles participate in the reaction, and what kind of bonds they need to form.
Yao plans to start his independent research group in 2024 and will focus on developing multimodal operando electron microscopy and synchrotron X-ray-based methods to elucidate the chemical dynamics of energy materials. The task is exactly the type of hands-on experimental chemistry that Yao enjoys.
And you can bet that movie-making will play a big role in it.