The Hunt for Dark Matter in the Universe
KICP's Juan Collar leads a team that detected a seasonal signal variation compatible with dark matter theory. In an interview, he discusses the significance of the finding, what will be needed to prove the existence of dark matter, and how turning to an old technology is helping scientists in the 21st Century.
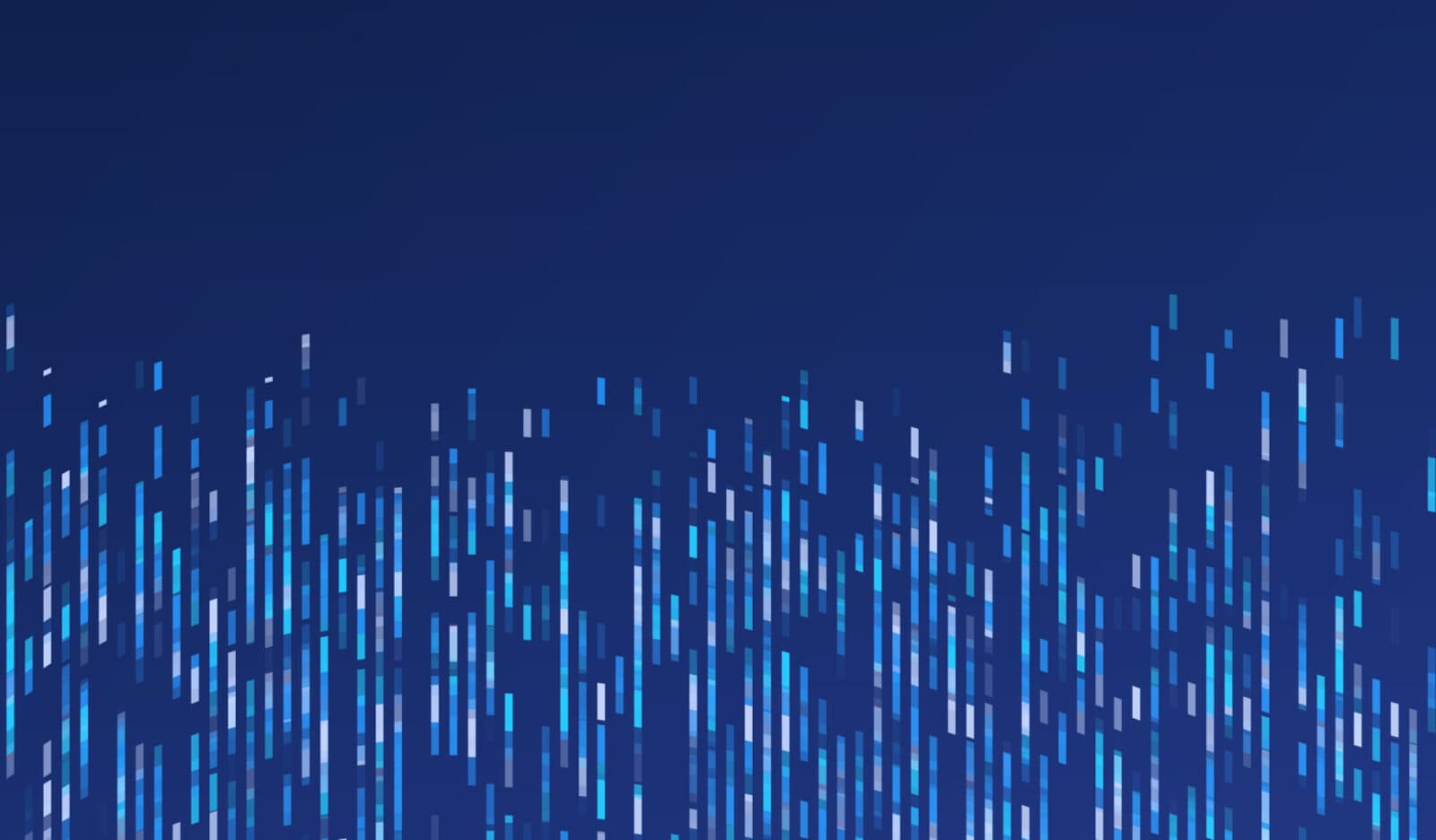
The Researcher
A DARK MATTER DETECTOR about 700 meters below the ground in a Minnesota mine has recorded a seasonal modulation in staggeringly faint electrical pulses – the possible result of dark matter particles called WIMPs that envelope the Milky Way galaxy and collide with atoms in the detector’s germanium crystal.

The finding, by the Coherent Germanium Neutrino Technology, or CoGeNT experiment at the Soudan mine, is described in a paper posted online by a team of researchers led by Juan Collar of the Kavli Institute for Cosmological Physics (KICP) at the University of Chicago.
The results are consistent with the modulation in signals first recorded more than a decade ago by the DArk MAtter/Large sodium Iodide Bulk for RAre processes (DAMA/LIBRA) experiment at Gran Sasso, Italy. It also appears to match recent but as-yet-unpublished findings by another experiment called CRESST, or the Cryogenic Rare Event Search with Superconducting Thermometers, also at Gran Sasso.
Collar said his experiment is carefully designed to detect the collisions between WIMPs (Weakly Interacting Massive Particles, which are hypothesized by dark matter theory) and atoms in the crystal. “It’s sensitive to very low energies, and that’s where we got stuck,” Collar said of CoGeNT’s detection of a modulation in signals. “We’re seeing something down there that we don’t quite understand yet.”
Collar and his colleagues, who have submitted the paper “Search for an Annual Modulation in a P-type Point Contact Germanium Dark Matter Detector” for publication, emphasized that the origin of the signals is unknown, but that the data collected from 442 days of observations “are prima facie congruent when the WIMP hypothesis is examined.”
Collar discussed his research, and the meaning of his recent findings, in a conversation with The Kavli Foundation.
A CONVERSATION WITH JUAN COLLAR
THE KAVLI FOUNDATION (TKF): Is your tentative result, which suggests a seasonal variation in the number of particle collisions with the nuclei in your detector, evidence for WIMPs – that is, evidence for dark matter?
JUAN COLLAR: You know, evidence is a big word in particle physics. In principle, one might be tempted to speak the language of evidence, but this whole situation with dark matter is so volatile one must be very cautious. We decided to share our data and publish. The impact is rather large, because our observations seem to agree with what DAMA has seen for about 10 years now. They are also similar to preliminary results from CRESST, another dark matter experiment.
So it's an important result. But evidence? We wouldn't touch that word with a ten-foot pole. There is evidence, perhaps, for a modulation in the data. But for the detection of dark matter? That is something that we’ve stated DAMA shouldn’t claim, even after 10 years of very solid observation of a modulation. The origin of this modulation does not necessarily have to be dark matter particles.
TKF: What is unique about your detector in the CoGeNT experiment, and what exactly is it detecting?
COLLAR: When a dark matter particle, a WIMP, strikes the nucleus of an atom in a germanium crystal, the nucleus recoils, striking other nearby atoms and knocking off their electrons. Our detector reads this ionization as an electrical signal. What's special about our detector is that it's optimized to look for light-mass dark matter particles, arguably the best for that particular job.

The reason for this is that most other detectors are designed to detect particles about 100 times the mass of a proton. But if WIMPS are much lighter than that, say just a few times the mass of a proton, then you need a detector with very low electronic noise. You have to be able to distinguish the very tiny energy depositions that are triggered by these collisions, because that's what you expect from lighter particles; they don't pack as much of a punch as something heavier.
That's what CoGeNT detectors are designed for, and that's where they perform very well, dramatically so. They are sensitive to very low energies, and that's where we got stuck. We’re observing something down there that we don't quite understand yet. We cannot yet tell you what it is, but of course we can list many possibilities. The low energy events that we end up with – we don't see anything anomalous in them. They look like they're produced by some form of radiation.
TKF: You detected a seasonal modulation in your data after 442 days of observations, and then your experiment was halted in March by a fire in the Soudan mine. Was the CoGeNT detector damaged? If it’s OK, will you continue to gather more data to strengthen your result?
COLLAR: It's a pure coincidence, but we started at the expected time for the minimum number of expected WIMP collisions. We started early in December of 2009, that is, we departed from the minimum, went through a full cycle, and on the way into the second cycle we ran out of luck. There was this fire in the mine shaft, and that stopped everything.
We were to some extent lucky. The underground laboratory is pretty large, and our side was untouched by either fire or fire-extinguishing fluid. That's what made much of the mess down there; so much foam had to be injected that it actually pushed the doors open to the laboratory and some experiments were completely covered by it. Our experiment was fine; but the detector went through a thermal cycle. Normally, we keep these devices at ninety degrees Kelvin, which is pretty chilly, and when you warm them up like this the quality of the vacuum inside the detector makes a critical difference. If it's a good vacuum, you should be fine when you turn it on again; if it's a bad vacuum, the detector could be useless for the delicate work we are trying to do. This is why we have analyzed the data collected up until the fire. We have 15 months of observations and we don't know if we'll have another day of good data with this device.
If this detector is not dead and the signal rate starts to decrease again toward next December and then picks up again afterward – the chances of seeing something like that start to become very small, very fast. The opposite side of the coin is, of course, if it doesn't do what I just described. In that case, the modulation we have observed so far may have been just a fluke.
TKF: One possibility is that you’re seeing a systematic error, that is, false signals that originate from the detector itself. But if CoGeNT, DAMA and CRESST – all different experiments with different kinds of detectors and different methods – are seeing the same seasonal modulation in collisions, isn’t it unlikely that the signal is some kind of systematic effect?
COLLAR: Indeed. Yes, you're right. The targets, or the material that the detectors are made of, are different. Also, each of these experiments collects and reads events differently.
"[It] gives you pause, the fact that the same kind of dark matter particle could be behind these three different observations from three very different detectors."
In our detector, particles strike the germanium crystal and produce ionization. In DAMA, particles strike sodium iodine, which is a scintillator material that generates light when radiation impinges on it, and that light is read out by a photomultiplier – an electronic eye of sorts – that turns light into electrical pulses. CRESST, meanwhile, is entirely different. CRESST uses a calcium tungstenate crystal, another scintillating material, but it is also operated as another type of detector called a bolometer, in this case, a hybrid bolometer. It’s a device where they read out the light that is produced through scintillation, but also the increase in temperature that is produced by the particle interaction.
So you're perfectly right, it's kind of suspicious that what these three experiments observe can be interpreted as a dark matter particle in common, of the same mass and with the same probability of interaction with different detector materials. That gives you pause, the fact that the same kind of dark matter particle could be behind these three different observations from three very different detectors.
TKF: The CRESST experiment appears to be seeing signals compatible with dark matter. What makes them confident that they are seeing something similar to what CoGeNT is seeing?
COLLAR: They've been exquisitely careful about trying to eliminate other possibilities. Over the last year or so, they have implemented different precautions, and have made different tests of their detector – all to exclude the possibility that what they're observing is a number of possible sources of background radiation. They've been discarding those one after another, and they're now left with about 20 events that they just don't know what to make of. They look like nuclear recoils, particularly affecting the oxygen in the crystal, and they cannot be explained away. They see evidence for 20 events that might be compatible with the same particle that might be producing a modulation in DAMA, or might be producing a modulation in our detector.
TKF: So, because the chance is very tiny that this is some background noise, the odds are higher that these collisions are being caused by dark matter particles, is that correct?
COLLAR: Well, that's not entirely true. The first part of the statement is correct. You can grow convinced that the chances are small that this is due to a background or any other non-exotic process that we can dream up. But it takes a leap of faith to go from there to the next step, claiming that what we are detecting is dark matter. That is actually why the community has been very critical of DAMA. They claim that the chances are tremendously small that their fluctuation is due to some kind of fluke. True. But going from there to stating, ergo it must be dark matter – that’s a hard call. But the DAMA collaboration seems not to be detoured by such considerations.
TKF: Could this modulation in collisions also be due to some kind of exotic physics that we don’t yet know about?

COLLAR: Well, exactly. It could be. It's one of three things: It's either dark matter, or it is something perfectly boring, systematic and instrumental – it wouldn’t be the first time in physics that we fool ourselves into thinking that something mundane is relevant. This sort of situation typically happens when agreement with other experiments is noticed, and soon after you are obsessed with observations that normally you wouldn't pay any attention to.
Or third, it might be something interesting that’s not related to dark matter. For instance, we could be noticing new effects arising from solar neutrino interactions in the detectors, as some phenomenologists are proposing. That would be pretty exciting, a new piece of physics not really related to dark matter. We’d still get to learn something about nature. Of course, the first possibility to consider is some instrumental effect that we have not figured out yet.
TKF: DAMA has claimed that they’ve also detected a seasonal modulation in their data, but other projects such as CDMS-II claim that they are not seeing compatibility with this modulation signal. What do you make of that?
COLLAR: CDMS-II is observing a spectrum of irreducible signals. That is, they’ve tried their best to reject them but they still remain, and they have essentially the same energy spectrum as ours. The material of their detector is also the same as ours – germanium.
CDMS can only marginally exclude what we are observing, and that is after much trying. I claim that they are not doing an unbiased job, and that they’re neglecting important facts. I don’t see any contradictions between CDMS-II data and ours. To the contrary, there is quite a good chance that there might be a lot in common between our data and theirs.
TKF: You've been involved in other experiments to detect dark matter particles – namely COUPP, the Chicagoland Observatory for Underground Particle Physics at Fermi National Laboratory. This experiment uses a quartz bell jar or bubble chamber as a detector for WIMPs. But this type of detector was conceived by physicist Donald Glaser in 1952. Why turn to an old technology in the 21st century race to detect dark matter?
The old saying “There's nothing new under the sky” actually applies remarkably well to the field of radiation detection. There are only so many ways you can detect radiation, and we seem to have gone through most of them. If you look at the history of our field, there have been very few important additions to the repertoire of radiation detectors in the last 30 or 40 years. There are instead a lot of variations around the same themes.
I started working with superheated liquids at the University of Paris. In my case, contact was purely coincidental. I was working on something unrelated to dark matter when I ran into a paper in a journal called Health Physics. They were talking about radiation dosimeters used to monitor neutron dose in hospitals, while being extremely insensitive to gamma radiation. I thought, “That sounds like a WIMP detector”. I went to my boss in Paris, Georges Waysand, and asked, “What's going on with these detectors?” and he said, “I have no idea.” So we decided to look into them. When I came to Chicago we moved to using the same concept in the form of bubble chambers.
"The old saying “There's nothing new under the sky” actually applies remarkably well to the field of radiation detection. There are only so many ways you can detect radiation, and we seem to have gone through most of them."
Let me explain something that is unique about the bubble chamber. In DAMA and CoGeNT, at the end of the day we are dealing with electrical pulses. In DAMA you have a flash of light that is invisible to the eye, and there's a photomultiplier that converts it and amplifies it into a small current. All these things are happening very, very close to the electronic noise in these devices. In CoGeNT, it's the same story. We pull the ionization signal out of the crystal and we amplify it. This is done close to the noise of the detector.
But a bubble is a bubble is a bubble. If you started to see an annual modulation in the rate of something that at the end of the day is macroscopic and can be detected via standard photography, there's no concern that there's some electronic noise or anything similar conspiring to give you signals you may mistake for a WIMP.
The whole beauty of the bubble chamber is that it all starts with the microscopic process of radiation interacting with matter. And you don't have to move a finger to amplify it. It's all coming from the fact that the liquid is out of equilibrium. It's an unstable system, and when an episode of microscopic boiling happens because a particle interacted with the fluid, everything that ensues, the formation of visible bubbles, is a spontaneous transition from the microscopic world to macroscopic observables. You don't have to amplify small signals through noisy chains of electronics or anything like it.
TKF: In bubble chamber detectors, WIMP collisions are expected to generate a single bubble, while other, more energetic particles are expected to trigger bubble tracks. Why is that?
COLLAR: When a WIMP strikes a nucleus, that nucleus doesn't go very far at all, whether the detector medium is a liquid or a solid. The distance is of the order of hundreds of angstroms, and not much more. The reason is that the nuclear recoil is pretty heavy. The nucleus slows down rapidly; it bangs around a lot and hits a lot of other nuclei, and it dissipates its energy very, very fast over a very short distance.

That's why when a nuclear recoil happens in a bubble chamber liquid, it produces a seed of nucleation, an episode of very local, microscopically localized high temperature, caused by this nucleus bouncing around and hitting other nuclei. If you were able to stick a thermometer at the point of interaction, it would measure an effective temperature in the hundreds or thousands of degrees.
Under some conditions, you can create a microscopic bubble. Those conditions are met for nuclear recoils because the local heating is very high, precisely because the nucleus doesn't go very far. The recoil track is very short. But for other types of radiation, the energy is deposited over much longer distances. It's the same amount of energy, but it is spread out, and the particle doesn’t heat up the liquid enough locally to produce bubbles. We fine-tune the temperature and pressure of the bubble chamber to be able to detect only bubbles produced by nuclear recoils like those expected from WIMPs, and not the tracks produced by uninteresting known particles.
We catch the bubbles in the act of forming when they are smaller than 1 millimeter. We take short movies, examining the frames in real time every ten milliseconds. The software is looking for a change in the image; essentially you have a motion sensor going, so you catch them as they grow. When you have confirmation that an image change is large enough to be a bubble, then you immediately recompress the liquid so that the whole volume doesn't boil, and you end up with a little movie covering a fraction of a second. In those you can see these bubbles start to grow, start to ascend through the fluid and then shrink back as we recompress the fluid. And then they disappear.
TKF: Getting back to the CoGeNT experiment, now that you’ve made public this result, what is the next step for you in the search for dark matter?
COLLAR: For about a year now, we’ve been designing the next generation of CoGeNT detectors, and hopefully they will have lower background noise and an improved ability to perceive smaller signals.
TKF: Is this the same detector model used by CoGeNT, with germanium crystals?
COLLAR: This is the same detector concept, but with a lot of improvements. It's essentially the same thing, but hopefully even better in performance.
TKF: What are your plans for continued studies with bubble chambers?
COLLAR: The bubble chamber, in principle, should allow us to test this hypothesis – that there should be a modulation in the rate of collisions between WIMPs and the nuclei in our detectors. We can make our bubble chamber sensitive to light WIMPs, and we have done that before. We have operated the chamber in conditions where we should be able to see the bubbles produced by these particles – if they exist.
The problem is that there are internal sources of neutrons in our bubble chamber. Some materials inside the bubble chamber are generating – at a very small rate – a neutron every so often. And we have evidence for that, because we see events containing multiple bubbles that can only be neutrons.
We identified where these neutrons are coming from, and we’re going to replace those parts of the detector this summer – these sources of internal neutrons in the bubble chamber. After that, we’ll attempt to look for light WIMPs with our bubble chambers.
TKF: What are the sources of these internal neutrons inside the bubble chamber?
COLLAR: In this particular case, they seem to be dominated by our ceramic piezos – they are electronic sensors that we use to detect the acoustic emission that accompanies the production of the bubbles. When bubbles form, it's a rather dramatic process. You have a liquid that is out of equilibrium. It’s super-heated, so it's a liquid that you've tricked into remaining a liquid when it should be a gas at the pressure and temperature you’ve set.
So, when a bubble forms, it yields quite a release of energy. The bubble expands very fast, and this produces a cracking sound that was described as a “plink” by the inventor of the bubble chamber many years ago – (in 1952, by physicist Donald Glaser at the University of Michigan). He actually used phonograph pickups to detect that sound in bubble chamber prototypes and trigger their photography. In our case, the sound is detected through the ceramic piezo-electric sensors, and we found out that there is enough uranium and thorium in them to produce these neutrons at a very, very low rate. We are getting a neutron essentially every week, and these neutrons start close enough to the active part of the chamber to give us some bubbles.
These piezos have to be replaced, and we already know how to do this. We found better ways to produce those ceramics. There are some inspection windows that need to be replaced as well. Those are the two main sources of internal neutrons that we could find. Everything else we think should be fine as it is.
TKF: So as long as you are eliminating all the known sources of noise, such as the ceramic material that you've identified in your bubble chamber, then hopefully what you're left with is an authentic signal of WIMPs, of dark matter?
COLLAR: Well…
TKF: That would be a leap of logic.
COLLAR: That would be a leap of faith. You would be left wondering, in the case of the bubble chamber, if you have rejected all the possibilities that your signals might be something else – for instance, some other background radiation.
"I'm going to quote my colleague here in Chicago, (astrophysicist) Rocky Kolb. Rocky says, ‘It's going to take a village to discover dark matter,’ and I agree with that. It's going to take more than the direct detection community observing these recoils."
Now, a few months ago, when the signals we have now identified to be these neutrons produced inside the chamber started to show, we had been pretty convinced that we hadn't missed anything. We thought, “These must be WIMPS”. And then we saw a triple bubble, which a WIMP cannot produce. WIMPS cannot interact more than once. You're lucky enough to get them to interact at all.
We immediately knew we had this neutron problem, and within a few weeks we knew where they had to be coming from. We measured the radioactivity in the piezos and other materials, and found agreement with the rate of neutron production. We explained away the observed signal.
There is a lesson to be learned there. It teaches you that, not only for bubble chambers but any other WIMP detector, after you clean things up you're always going to be left wondering what else it is that you missed. Particle physics and in particular dark matter searches can be very tricky.
TKF: Another approach on the road toward detecting WIMPs, besides measuring the seasonal modulation, is to measure the direction that a nuclei recoils after a particle collides with it – and the modulation in the direction of recoil over time. That’s because according to dark matter theory, WIMPs are expected to strike the Earth from a particular direction in the sky, depending on the time of the day. How far are we from making this kind of measurement, and would it provide stronger evidence for dark matter?
COLLAR: That's actually a technology that nobody has yet – a technology that can see the direction of the recoil. There are small prototypes that researchers have been building, and it is an extremely promising technique.
What we’re noticing is just a modulation in the rate of collisions, and that’s a lot less complex than detecting a modulation in the direction of the recoil. So right now, what we and everyone else who is trying to detect dark matter have available is technology to look for this annual modulation in rate. That's a poor man's smoking gun, unfortunately.
The day someone observes the modulation in the direction of the recoils – that would be very hard to mimic, and it would be the next step on the road to a discovery. It would be very hard to explain such data as anything else other than dark matter, because there's essentially nothing else in nature that could imitate that.
The problem is that the only way that we know how to spot the direction of a recoil is by stretching the short tracks they make in a solid. They only span on the order of 500 angstroms, so there's no way we could image that in a radiation detector. The solution, then, is to rarify the detector medium, to move to gaseous detectors and to operate them at very low pressure. Then, those tracks – those little tracks that the recoils might produce with a preferred direction if they're coming from WIMPS – they can be stretched to a few millimeters. We have the technologies to image that – barely, but we do.
So, the challenge is that your detector has to use targets that have a very low mass because their density has to be very, very small. At the same time, the detectors must have enough overall mass in order to interact with incoming WIMPs, which have, by definition, a very low probability of striking. And then you end up with enormous devices at a very high cost. One day we may be able to observe this beautiful WIMP signature, but right now the detectors are just small prototypes.
TKF: Let’s say eventually you are able to confirm this seasonal modulation in collisions with CoGeNT and with COUPP, and other different experiments show the same thing. And then the day arrives when we can measure the direction of recoils, and we detect a daily modulation in the direction of recoils.
If all these results are replicated with different experiments around the world, which use different materials and different methods, would you feel confident that we have detected WIMPs – that is, dark matter?

COLLAR: I'm going to quote my colleague here in Chicago, (astrophysicist) Rocky Kolb. Rocky says, ‘It's going to take a village to discover dark matter,’ and I agree with that. It's going to take more than the direct detection community observing these recoils. Certainly the directional signature would be fantastic, but I think we’re really far away from getting to that point, if we ever get to that point.
If several experiments of our kind detect the same modulation, that would be tremendous. But personally, I believe you even need a bit more than that. And what else is that little extra?
Well, accelerator experiments are in principle sensitive to the same types of particles that we’re searching. If you can produce man-made WIMPs in accelerator experiments, and they match the properties that we observe in our experiments, that would be fantastic.
On top of anything that the detection community might find, there's a theoretical framework that explains why these particles exist, why they have the mass they have, the probability of interaction that we observe in experiments, etc. This theoretical framework also generates new predictions that you can go out and test.
If those theories are confirmed, at that point, we could say that we did it.
TKF: What are the perils of relying solely on direct detection experiments, without returning to theory to check on what you are seeing?
COLLAR: The problem with just relying on direct detection experiments is the following: we are biased. For instance, we are paying attention to these light WIMPs, but why? Because they are really almost the only thing left that could explain DAMA’s observations. Everything else seems to be excluded, so you start to pay a lot of attention to those.
Now, maybe the CRESST guys are paying more attention to those 20 events than they should, because they're biased by DAMA’s pre-existing results. Same exact thing applies to us and our modulation within CoGeNT. So on and so forth.
There have been plenty episodes in the history of particle physics where a number of experiments have observed the same thing, and then years later an equally large number of experiments found nothing. And in some instances, there was never a good explanation as to why the first batch of experiments saw anything at all in the first place.
You know, things come and go. A few years ago, physicists thought they were observing nuclear structures containing five quarks, the so-called pentaquarks. Eleven experiments had pretty good solid evidence for them, and then many more experiments came along and saw nothing. And now the interest is pretty much dead.
Nobody knows what happened there. We do not have a good explanation as to why these objects were observed in the first place. The evidence just went away. So that's why you should not rely exclusively on direct detection experiments for dark matter, in my opinion.
I am much more conservative than many of my colleagues. Some will tell you that their experiment, on its own, is going to find dark matter, with absolute certainty. Crazy as that sounds.