Disturbing the Nanosphere
Cornell University researchers deliberately created atomic-level disorder in order to probe the workings of heavy fermion compounds. Could this experiment revolutionize 21st century technology?
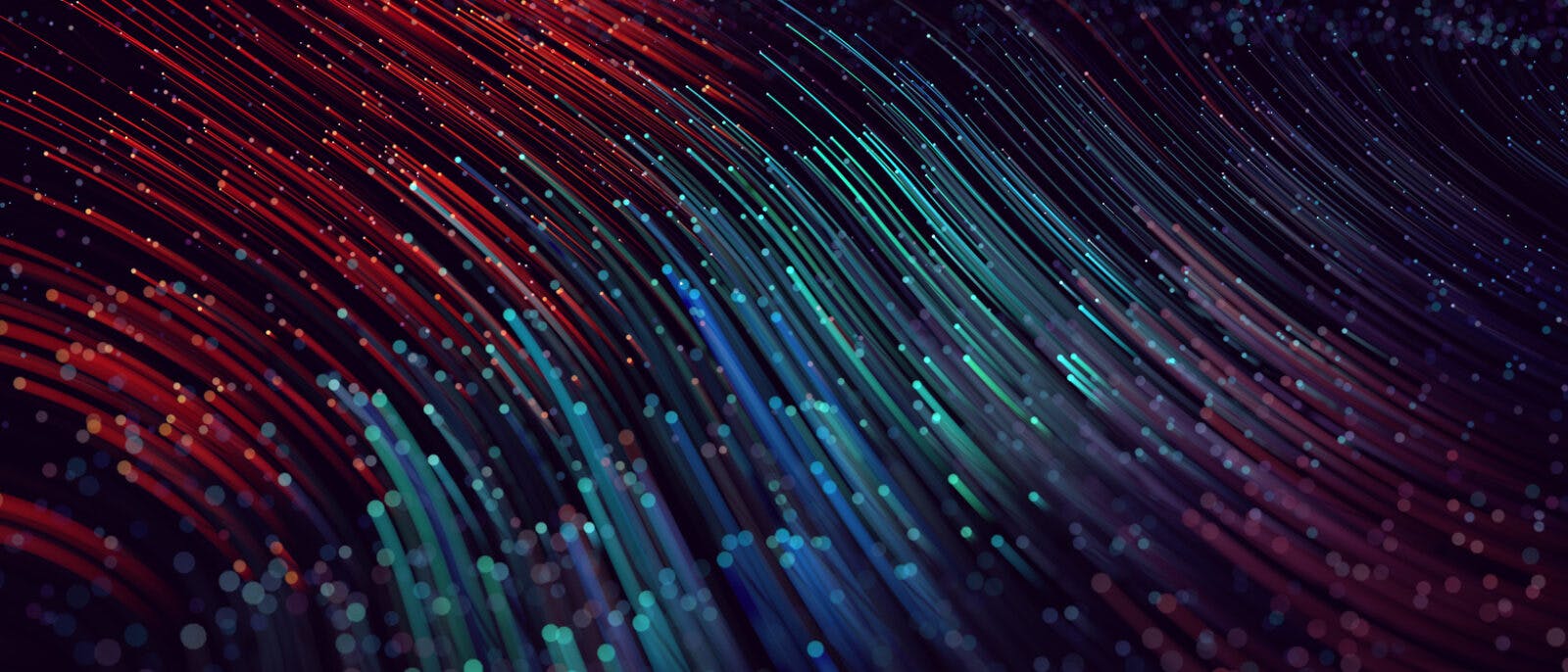
The Researcher
NEW IMAGING TECHNOLOGY is giving scientists unprecedented views of the processes that affect the flow of electrons through materials. In one such experiment, a team at Cornell University’s Laboratory for Atomic and Solid State Physics has altered a familiar tool in nanoscience, the Scanning Tunneling Microscope, to probe for very tiny energy variations. By using the microscope for “Spectroscopic Imaging,” scientists were able to visualize what happens when they change the electronic structure of a “heavy fermion” compound made of uranium, ruthenium and silicon.
What they saw was widespread disorder – and, with it, clues to the physics of magnetism and conduction. This knowledge also sheds light on superconductivity, the movement of electrons without resistance, which typically occurs at extremely low temperatures and could revolutionize electronics if scientists can find a way achieve it at something close to room temperature.
The Cornell experiment and its results are presented in the Proceedings of the National Academy of Sciences (see “How Kondo-holes create intense nanoscale heavy-fermion hybridization disorder,” PNAS, available online). The research team included J.C. Séamus Davis, a member of the Kavli Institute at Cornell for Nanoscale Science and developer of the SI-STM technique. Working with synthesized samples created by Graeme Luke from McMaster University (Canada), the experiment was designed by Mohammad H. Hamidian, a post-doctoral fellow in Davis’ research group, along with Andrew R. Schmidt, a former student of Davis at Cornell and now a post-doctoral fellow in physics at UC Berkeley. Inês Firmo, a graduate student and Milan Allan a postoral associate in the Davis lab contributed to the new analysis techniques developed for this project.
Hamidian recently talked with The Kavli Foundation about the heavy-fermion research, its significance to condensed-matter physics and the study of superconductivity, and the exciting possibilities opened up by Cornell’s Spectroscopic Imaging-Scanning Tunneling Microscope (SI-STM). Here are highlights of that conversation:
THE KAVLI FOUNDATION (TKF): Let’s begin by discussing heavy fermions. In this case, heavy fermions are electrons that seem “heavy” because they’re slowed down by intense interactions with magnetic atoms in the material they’re travelling through. What is it about heavy fermion systems that make them so interesting?
MOHAMMAD H. HAMIDIAN: Heavy fermions provide a platform to study two big issues in condensed matter physics, which are also applicable to the technology of the 21st century. One is magnetic interactions at the atomic scale, and the other is achieving superconductivity through unconventional means.

TKF: So magnetism could be a switch to control superconductivity.
HAMIDIAN: Yes. One reason we study high temperature superconductorsis to find out what causes the transition temperature of superconductivity to be as high as it is. And knowing that, perhaps we can tune the temperature so it can be even higher.
TKF: And if you do that, what implication does that have for, say, computing?
HAMIDIAN: Many of the devices that people are thinking about using in the future would use superconducting circuits. You may have heard the term “superconducting qubit.” These are circuits that have superconducting components in them, but at the present time the only feasible superconductor that can be used is a conventional metal, and you can only make it superconducting at very, very low temperatures – liquid helium temperatures, actually. To make it feasible to eventually use these superconductors in a desktop computer, you have to be able to drive up the transition temperature from very close to absolute zero to room temperature. And to do that, you really have to have an understanding of how the material works.
TKF: So what did you do in this experiment and what did you find?

This is interesting, because the presence of magnetic atoms is typically detrimental to electron flow and conventional superconductivity. In this case, however, we found that removing the magnetic atoms proved detrimental to the flow. In other words, by creating a “Kondo hole,”, the elimination of the Kondo Effect at a given atom, we took away a very essential element of what makes a heavy fermion. The heavy fermion needs to be able to switch between states, and when suddenly electrons could no longer attach to the magnetic atoms, this sent out ripples into the rest of the material, forcing other electrons to change their character as well. In effect, the glue that held the heavy fermion electrons together was severely weakened by a very small number of Kondo holes across the entire material.
TKF: Which reveals that heavy fermion material, which might be critical for understanding high-temperature superconductivity, won’t work without this magnetic relationship – even though with other substances, this would typically be detrimental to superconductivity. So, you uncovered this by specially modifying a scanning tunneling microscope?
HAMIDIAN: Yes. A scanning tunneling microscope is not a microscope in which you use light to see the atoms or to see the electrons; it pulls or pushes electrons into the material. With a new technique, we are now using the microscope for measuring how hard it is to push in and pull out an electron at a given spot in a heavy fermion compound. By doing this, we actually learn a lot about the material’s electronic structure. Then by mapping that structure out over a wide area, we can start seeing variations in those electronic states, which come about for quantum-mechanical reasons. Our newest advance, crucial to this paper, was the ability to see, at each atom, the strength of the interactions that make the electrons ‘heavy.'
So how could that lead to anything useful? Well, if you know something about the quantum-mechanical state of a material and the electron flavor, you know a lot. In fact, you know how the electrons move at a given energy, you know what momentum they have, you know how the system has self-organized to lead to those particular values and how it can be tuned by introducing defects.
TKF: Where does your research proceed from here?
HAMIDIAN: The paper’s main point was that Kondo holes have a destructive impact on these heavy fermion materials. More importantly however, was developing new SI-STM technology and techniques that enabled us to study this very rich physics . This was an incredibly difficult project, because the energy variations that you have to be able to probe are very tiny; but we now have the ability to even measure the strength of the “glue” which holds heavy fermions together. Ultimately, we are trying to link heavy fermions and superconductivity in a much more solid and comprehensive way. By continuing to develop the SI-STM we hope to open up a whole new channel of atomic-scale study for complex materials that will form the basis of technology in the coming century.