Dancing in the Dark
by Alan S. Brown
Nanoscience enabled Nynke Dekker to investigate individual DNA and RNA molecules. Now she wants to understand how they behave when they replicate
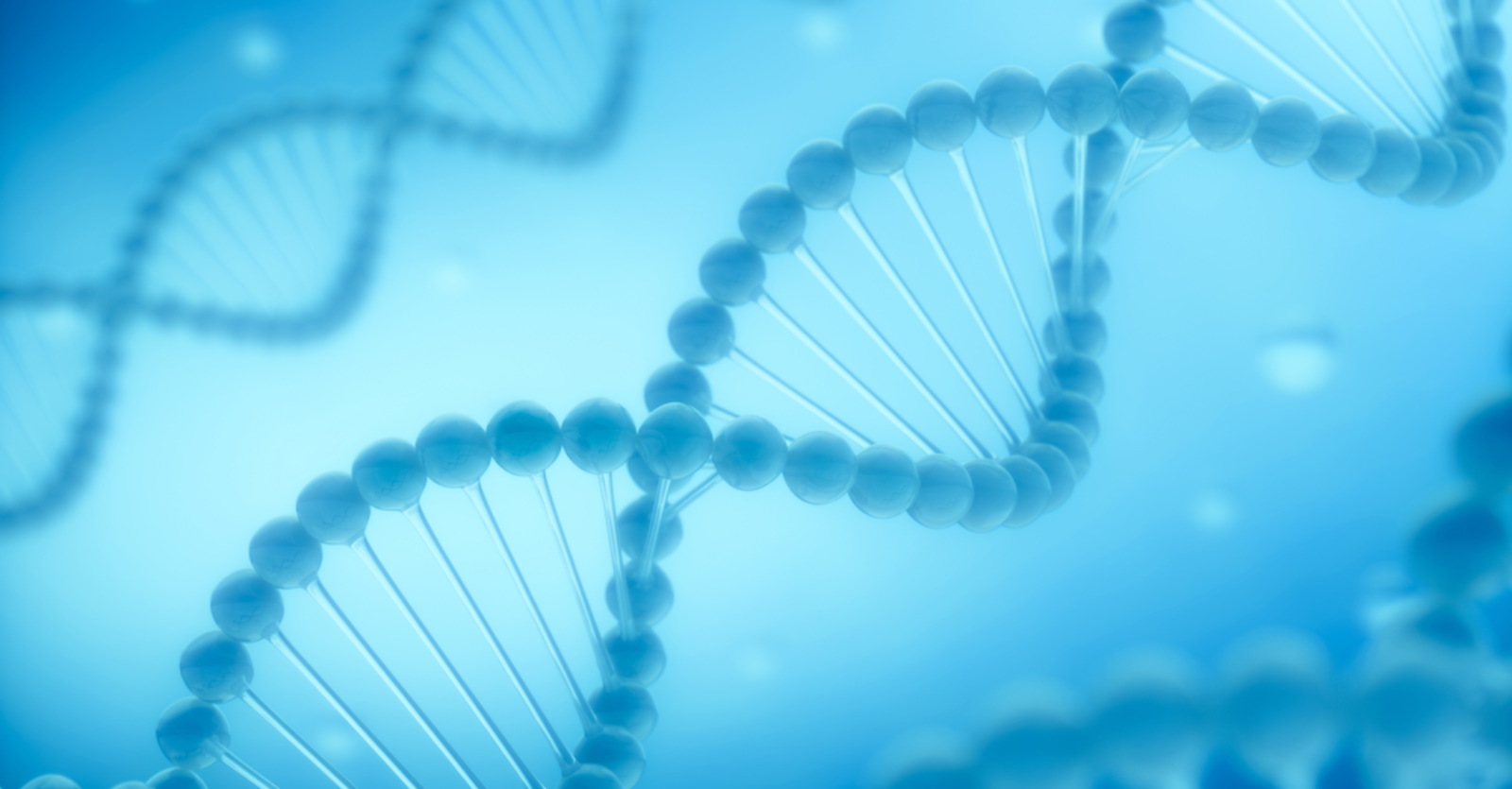
The Author
The Researcher
Imagine watching a ballet in the dark. The dancers wear dabs of fluorescent paint—colors based on their roles in the dance—that glow when struck by laser light. This lets you track each color’s location, how long it stays there, and the other colors near it. Using only that information, your goal is to visualize the jumps, spins, and lifts taking place on stage.
Now, imagine doing this with molecules at the nanoscale, where thousands of dancers could line up across a stage the width of a human hair. This is the challenge Nynke Dekker faces as she probes how proteins copy DNA inside cells.
Dekker, a member of the Kavli Institute of Nanoscience at Delft University of Technology, is this year’s recipient of the Spinoza Prize, one of The Netherlands’ two most prestigious science honors. The award cites her pioneering studies of individual DNA and RNA molecules and how they interact with proteins. Her findings may one day allow us to design drugs that stop infectious viruses and cancer cells from replicating.

Her current research looks at how different types of proteins work together to replicate DNA molecules within yeast cells. Like our imaginary ballet viewer, she tags proteins with fluorescent molecules, lights them up, and then tries to interpret—with the help of software models—how their intricate dance plays out as they unravel, cut, splice, copy, and coil DNA.
It has taken Dekker 20 years to learn how to make sense of these dancing proteins. But first, she had to learn how to study individual DNA and RNA molecules.
Instruments
Although Dekker was born in The Netherlands, she grew up in the United States and entered Yale as a biophysics and molecular biochemistry major. That changed when she found her chemistry lab filled with pre-med students who cared more about grades than science.
“I didn’t really like their approach,” she recalled. “But in physics lab, I could play with wires and instruments and it was more fun.” She kept at it and eventually went for a doctorate in atomic physics at Harvard.
As she neared the end of her studies, however, she began to feel restless. Atomic physics, she felt, was well-understood science. Her Ph.D. project, for example, involved calculating ways to steer cesium atoms on a chip, then building an apparatus to do it. “It was cool to demonstrate it could be done, but it was not a surprise that it worked,” she said.
Dekker wanted the unknown, a field where researchers had not yet nailed down the fundamentals. By then, the Human Genome Project had begun to deliver its first results. She saw that biology was growing more quantitative and driven by the type of computer modeling she did as a physicist.
This drew her to biophysics. “I was totally naive about the research,” she said. “I thought it would be fun to use my knowledge of physics to contribute to biology, though I don’t think I could have articulated how I would do that.”
After receiving her doctorate, she took a two-year post-doc with David Bensimon and Vincent Croquette of École Normale Supérieure in Paris. They were pioneers of single molecule biophysics, developing instruments to hold, prod, and twist single strands of DNA—and to measure the results.
By the time Dekker joined the lab, they were studying how those twists and pulls changed how DNA interacted with proteins. They used the data from those experiments to build computer models that provided new insights into DNA behavior.
Dekker began working with DNA topoisomerases, proteins that help shape DNA by coiling and uncoiling parts of the strand. This involved using a variety of specialized instruments, many of them pioneered in the lab, to manipulate individual molecules.
One of them was the magnetic tweezers, used a magnet to twist or pull a metal nanosphere that she had previously bonded to the DNA molecule.
“I still remember the first time I pulled on a DNA molecule,” she said. “It was so cool, just because you could actually do something like that.”
She also worked with other advanced instruments, like optical tweezers, which move around—and even levitate—molecules with focused laser beams. She also learned to tag molecules with fluorescent “labels.”
Dekker became a tool builder herself. She helped develop a more precise magnetic tweezer and, after she had set up her own lab at Delft, built a unit that measured the forces on hundreds of DNA strands at once.
I still remember the first time I pulled on a DNA molecule. It was so cool, just because you could actually do something like that.
Nynke Dekker
Understanding DNA
At Delft, Dekker’s tools enabled her to do explore DNA and RNA in ways that had never been done before. Her early work, for example, provided new insights into chemotherapy drugs, showing how they slowed the activity of topoisomerase that coiled DNA for reproduction.
She also investigated RNA polymerases, proteins that synthesize RNA from a DNA template. As she learned more about the behavior of the polymerases, Dekker began adding antiviral drugs to her samples. This stalled or impaired their ability to make RNA. Researchers are now using this technique to understand how potential Covid-19 drugs, such as remdesivir, work at the molecular level. Insights from this work may help researchers tune an existing drug to make it more effective or design a new drug altogether, she said.
In landmark research, she showed how virus proteins insert errors in RNA, and how antiviral inhibitors can disrupt these proteins. She investigated cells so stressed, they had compacted their DNA until it was almost crystalline, yet they were still able to create RNA, which carries instructions to manufacture proteins the cell needs to live.
After years of working with single molecules and individual proteins, Dekker pivoted to study how proteins work together to prepare DNA for replication. It is an enormously complex process.
“In cells like ours, you need something like 20 or 30 proteins working together to do it,” she said. It has taken four years, but her team is now working with about 10 proteins at a time, labeling each type of protein with its own colored fluorescent molecule. Using magnetic tweezers to suspend DNA in water, she films the proteins under a powerful optical microscope using a high-speed camera.
The team then uses a computer to interpret the camera’s colored blurs. They not only track individual proteins, but the order in which proteins go to a location and which other proteins are present around them.
It has been a long journey, but Dekker’s team has begun to make sense of the molecular ballet playing out on the stage, the solos and the pas-de-deux.
“I’ve arrived at the stage where I’m finally doing molecular biology, like I wanted to major in when I started school,” she said. “Now I want to integrate the physics I’m doing with other approaches developed to understand what goes on in cells, like genetics, bioinformatics, sequencing, and things like that. And to understand not just when everything goes right, but why things go wrong and why cancer cells can handle them better than our cells.”
It is an agenda that will keep Dekker busy for decades, even if she is just watching molecules dancing in the dark.