DNA Traces Disease Back to Its Origin
by Alan S. Brown
Billions of DNA fragments are circulating in our bloodstream. Now, researchers can use them to trace a disease back to its source
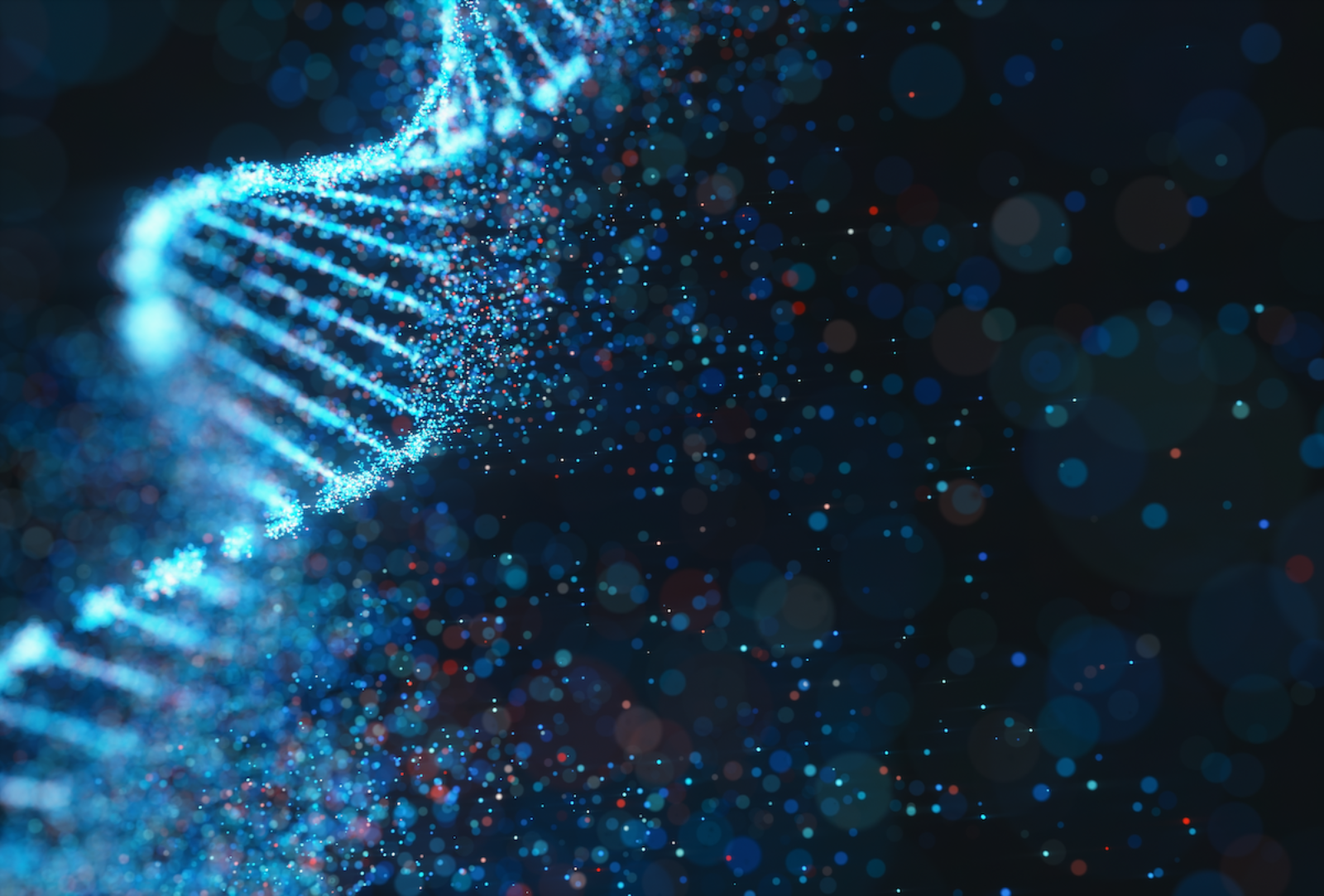
The Author
The Researcher
The typical human body has more than 30 trillion cells divided among at least 200 different cell types. Yet all of them have one thing in common: they share the same genome.
When those cells die — most cells live only a few weeks — some of that DNA ends up in the bloodstream. This is especially true if they die from disease or injury. Once in the blood, circulatory system enzymes chop the long strands into short fragments. So much DNA circulates through our bodies that a single milliliter of plasma might contain 10 to 100 billion segments.
There is a lot of data locked in these fragments, and for many years, scientists speculated about what secrets they might hold about our health. Thanks to high-throughput sequencing, we now have the means to decode their composition very quickly. Yet if every cell’s genome in the body is an almost perfect replica of the other, what could they possibly tell us?
Quite a lot, says Iwijn De Vlaminck, an associate professor at Cornell University’s Meinig School of Biomedical Engineering and a member of the Kavli Institute at Cornell for Nanoscale Science. In fact, by understanding how different types of cells and organs use the same DNA differently, De Vlaminck has found a way to trace those DNA fragments back to the organ of their origin.
This is not a mere academic exercise. Too many fragments from a particular organ or tissue can tell physicians if a patient is ill and what organs are affected. De Vlaminck has used this “liquid biopsy” technique to diagnose the progression of COVID-19 through the body’s organs and uncover surprising links between the Coronavirus and the stem cells that produce red blood cells. His lab has also used free-cell DNA to diagnose bacterial infections and to provide an early warning when the body starts to reject an organ transplant.
This is only the start. Diagnostics based on cell-free DNA could warn of cancer before it is visible any other way and provide feedback on how well a particular treatment is working. It could also signal a variety of genetic and bacterial diseases well before patients start showing symptoms.
“You could easily imagine a world five or 10 years from now in which our cell-free DNA tests will be part of regular blood workups,” he said.
Marrying technologies
De Vlaminck’s route to liquid biopsies was convoluted. While earning a Ph.D. in nanotechnology and applied science at Katholieke Universiteit Leuven in Belgium, he grew interested in using nanodevices as biosensors. This led to a postdoctoral fellowship with Cees Dekker, then co-director of the Kavli Institute of Nanoscience at Technical University of Delft in The Netherlands, where he learned to trick measurements out of individual molecules. Then he joined Stephen Quake’s lab at Stanford University, where he investigated the use of cell-free DNA for blood tests.
The first problem De Vlaminck faced was finding a way to make sense of all the DNA fragments in the blood. All DNA is made up of just four nucleic acids, which are rearranged along a strand of DNA in a mind-numbing number of ways.
Fortunately, De Vlaminck was riding a revolution in high-throughput sequencing, devices that automatically measure the composition and order of those nucleic acids. Sequencers have grown much faster and much less costly over the past few years. That gave De Vlaminck a way to map those billions of DNA fragments in each sample.
Yet, if all the cells in the body shared the same DNA, what information could he possibly extract from them? Starting in the 1990s, researchers realized there was at least one case where the blood might carry fragments of DNA from a different donor — when a woman was pregnant. The DNA of a fetus is an amalgam of its mother’s and father’s DNA, and some of it finds its way into the mother’s bloodstream.
Cell-free DNA testing could warn families if the fetus showed signs of Down syndrome, trisomy 18, spina bifida and other genetic diseases. In the past, a woman who wanted that information had to undergo amniocentesis, a procedure that involved puncturing the amniotic sac and that was potentially dangerous to the fetus. In 2012, after years of testing, the American Congress of Obstetricians and Gynecologists approved a non-invasive free-cell DNA test for women with high-risk pregnancies.
De Vlaminck found another exception—patients with organ transplants. These genetic differences are easily targeted because transplanted organs have an entirely different genome. In fact, patients must take a variety of pharmaceuticals to keep their immune systems from rejecting those foreign organs. Sometimes, those treatments are not fully successful, and the body will begin to attack the transplant.
In the past, patients showing signs of rejection underwent a conventional biopsy. Surgeons removed small sections of a transplanted heart, lung, liver, or kidney and looked for signs of rejection under a microscope.
“With cell-free DNA, you can assess injury from the blood without an invasive procedure,” De Vlaminck said. “We have shown that we can use DNA to potentially quantify an injury. The results are not yet as good as a traditional biopsy, but it’s much easier on patients. But then we’re back to this problem—most patients are not solid organ transplant patients. Most of their cell-free DNA is coming from their own bodies. So, how do we figure out which organs and tissues it’s coming from so we can diagnose a problem?”
Tracing COVID-19
The answer lay in the emerging science of epigenetics. While the body’s cells might all harbor the same DNA, epigenetics examines how environment and behavior change how cells use that DNA. It seeks to explain why, for example, liver tissues develop differently than heart muscles and why some cells make certain proteins but not others.
On a cellular level, epigenetics looks at the switches that turn genes on and off. Cells and tissues do this in many ways including a simple chemical process called methylation, which leaves behind very slight chemical modifications of the DNA itself. These methylation markers are specific to each type of organ and tissue in the body, and they can be identified in cell-free DNA.
By identifying and counting the methylation markers, De Vlaminck could now trace the blood-borne fragments back to the specific organ or tissue from which they came. The more cell-free DNA from a specific site, the more likely it had suffered an injury. For the first time, he had a way of assessing injury in a patient.
De Vlaminck was ready to test that approach when COVID-19 swept across the globe in early 2020. Physicians knew that the Coronavirus caused lung injury, but patients were showing other symptoms as well. Post-mortems showed the virus could affect many different organs, including kidneys, liver, heart, and intestines.
De Vlaminck thought his blood test might allow hospitals to use information about infected organs to decide which patients required critical care. It could also give physicians a way to gauge if their treatments were improving the health of those organs.
To see if this would work, De Vlaminck set up collaborations with Dana-Farber Cancer Institute, McGill University, and University of California, San Francisco. The medical centers procured plasma samples from patients with severe infections for De Vlaminck to analyze. He sent the results back to the hospitals, which compared them with the patients’ clinical records.
Some results were expected. Most patients suffered lung injuries, and they often showed liver and kidney damage. Unexpectedly, De Vlaminck often found indications that the virus was affecting the production of red blood cells.
“For sicker patients, we found a lot of DNA derived from red blood cell progenitors that produce red blood cells in our bodies,” he explained. “This indicated a direct involvement of the virus with the greater turnover of these cells. Also, that increase scaled very strongly with the severity of the disease.”
There are many potential explanations of why this happens. The Coronavirus might attack those cells directly, or it could put on stress the immune system and cause a faster turnover of cells. Either way, it opens a door to future research and a clearer understanding of the virus that can help medical professionals combat the most severe cases.
De Vlaminck also wants to use similar blood tests to investigate other COVID-19 outcomes, like patients who struggle with symptoms for months and multi-inflammatory syndrome in children (MISC). “It would be useful to see what is different about these subjects and perhaps that will lead us to new treatments and new therapies,” he said.
Liquid biopsies
Meanwhile, De Vlaminck continues to pioneer new uses cell-free DNA methylation markers. He was the first to show that it could identify bacterial infections by matching bacterial DNA with that of an infected organ. His group is also investigating bone marrow transplants.
Despite the success of the COVID-19 study, De Vlaminck thinks the new technology needs work. A growing number of labs across the world are doing that right now. It may take five or 10 or 15 years, but De Vlaminck sees many potential applications.
Cell-free DNA could be used to screen for cancer. “Cancer is a disease of the genome, where a mutation causes a cell to grow faster than the cells around it,” he said. “These mutations are well known, they happen in reoccurring specific genes, and they release lots of DNA. You could use it to detect early cancer by looking for these telltale mutations. You could also use it to evaluate whether a therapy is working or not, where the tumor is shrinking, or whether the tumor is recurring. And you don’t have to do an intrusive tissue biopsy to get this information.”
The technique should work on other immune system diseases, such as inflammatory bowel diseases and bacterial infections. Many researchers are also looking at cell-free DNA in urine to monitor urinary tract and other infections, including those that are ordinarily difficult to diagnose.
De Vlaminck’s data-centric approach generates a lot of very deep genetic information about the health of subjects. This is the type of data that machine learning can sort through to look for DNA signals that might predict some diseases even further in advance, giving physicians even more time to intervene before symptoms begin to show.
“The earlier you can identify that something is going wrong, the more time we have to intervene before the disease progresses,” De Vlaminck said. “This is true for cancer and for almost everything else. Cell-free DNA may ultimately give us a way to do this, because today, we’re only scratching the surface of its potential.”