Doing the "Impossible"
by Alan S. Brown
Research highlights from Kavli Nanoscience Institutes
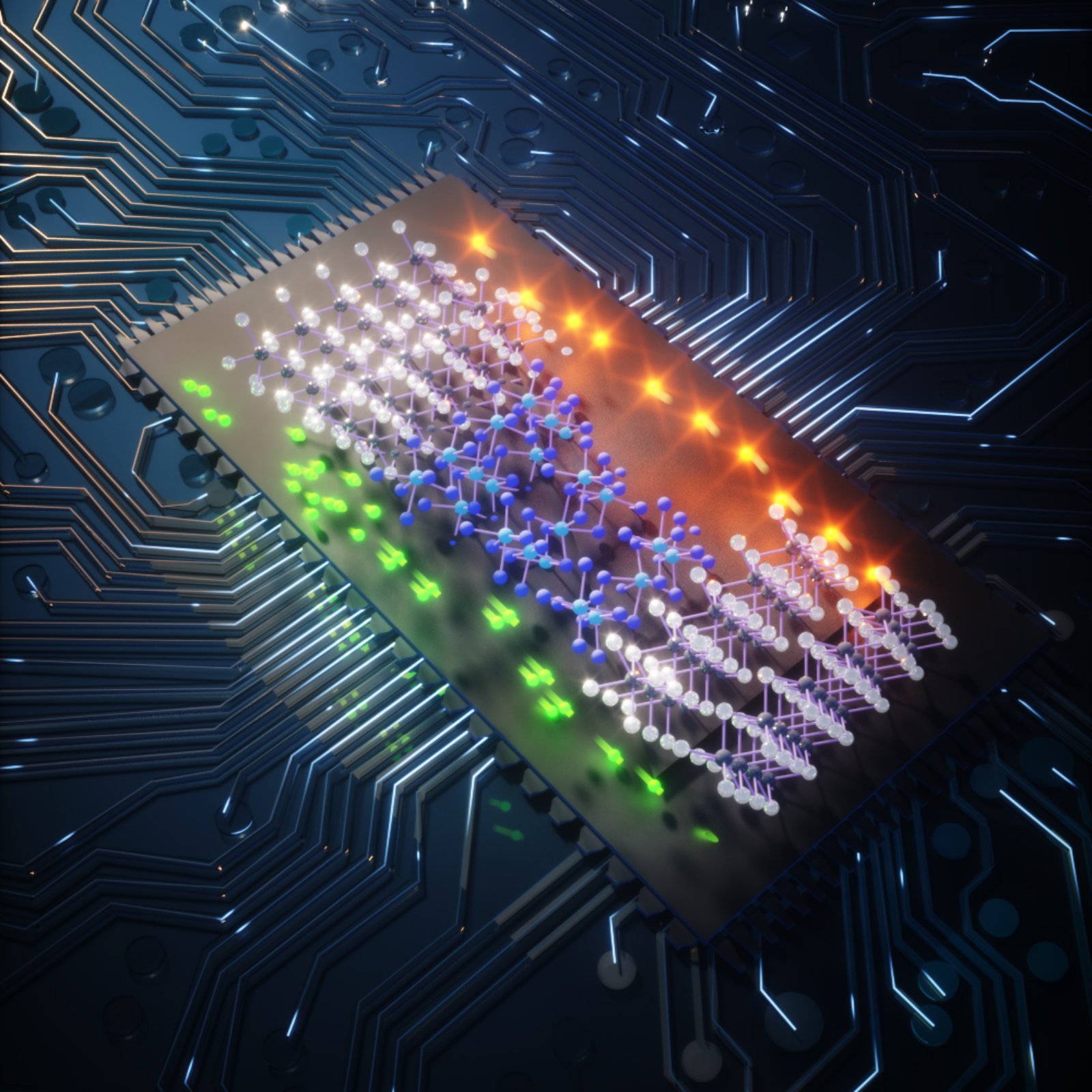
The Author
May was a fabulous month for nanoscience breakthroughs. Let’s start with the first quantum network to use entanglement to exchange information between unconnected nodes. This demonstrates the technology needed to create a true, ultra-secure quantum network. Then comes an “impossible” superconductor that conducts electricity in only one direction without manipulating its magnetic field. This is the equivalent of an electronic diode and could open the door to functional, all-superconductor circuitry. Then we move onto the first demonstration of a way to accelerate a magnet’s up and down electron spins at different velocities. If that were not enough, we also discuss a new platform that—for the first time—lets researchers investigate coupled materials systems that do not conserve their total energy when they transform. And finally, we look at individually controlled nanowires that can emulate the body’s cilia, splitting and recombining streams of liquids or even creating whirlpools.
Quantum network breakthrough skips a node.
For the first time, a quantum network has exchanged information between non-neighboring nodes. Essentially, the data “teleported” from node C (for Charlie) to A (Alice) without going through B (Bob). The work, led by Ronald Hanson, a member of the Kavli Institute of Nanoscience at Delft University of Technology, demonstrated the practical architecture needed to build much larger network that will be able to transmit data far more securely than today’s internet. The keys to the new architecture are innovations in quantum bit (“qubit”) management that preserve the remote entanglement that links the two qubits. Specifically, Hanson’s team solved the problem with innovative qubit readout procedures, by creating memory qubits that held information long enough for Alice to entangle an electron spin with Bob, who shared that entanglement with Charlie. When Hanson changed Charlie’s quantum state, Alice’s state changed as well without passing through Bob. The fidelity of the teleportation was improved by an innovative “heralding” procedure. This type of entangled network would enable computers to send a security code to one another without information traveling over internet cables.
“Impossible” superconductor conducts in one direction only.
Imagine yourself skating smoothly over an ice rink, then turning around and finding yourself unable to skate in the other direction. It sounds impossible, yet researchers led by Mazhar Ali, a member of the Kavli Institute of Nanoscience at Delft University of Technology, have done exactly that with superconductors. The latter are materials that conduct electricity without any resistance. Theoretically, a superconductor-based computer given a single jolt of electricity could continue to run without any adding additional current. The material’s properties resemble those of a one-way semiconductor diode, an essential component of electronic circuitry. Superconducting circuitry could potentially replace conventional electronics in quantum computers, since they would operate better at near absolute zero temperatures and not produce heat (due to electrical resistance) that cause qubits to destabilize. While others have found ways to make one-way superconductors, this is the first time it has been done without cumbersome manipulation of the material’s magnetic fields. The researchers stumbled onto the material while investigating thin films of niobium bromide. They are still trying to understand why it behaves the way it does.
Split electron spins could lead to better memory.
Researchers have long pursued magnetic memory because it can hold data for extended times. To compete with its silicon, however, it must grow denser and more energy efficient. 2D magnets (based on atomically thin layers of materials) promise better performance for electronics, but first we need a better way to flip the currents created by aligned electron spins from up to down, thereby changing the field’s orientation (the equivalent of turning a transistor on an off). Most researchers do this with an electrical field, but not a team led by Dan Ralph, a member of the Kavli Institute at Cornell for Nanoscale Science. Instead, the team used antiferromagnetic materials, which have no net magnetic orientation because every adjacent electron spins in an opposite direction. When the angle of the antiferromagnet aligns properly with a soft ferromagnet (nickel-iron Permalloy), it can actually split the Permalloy’s up and down spins. Surprisingly—split spins have been predicted but never demonstrated—this accelerates one set of spins faster than the other. By controlling the angle of the antiferromagnet to the ferromagnet, they can also switch more efficiently than other approaches. Now, the team is looking for ways to take advantage of these properties to make unique materials—and, perhaps, nonvolatile memories that are both efficient and very dense.
Looking at leaky couples
A new way of looking at how transformations in the shapes of materials coupled to one another alter system properties could lead to advances in such fields as electronics and photonics. This topic of coupled systems, a branch of topological physics, has been studied for years and has already led to some key breakthroughs. But studies in the past have focused on what are called “conservatively coupled systems.” These are systems where changes do not change the total energy in the system, and they are relatively straightforward to study. In the real world, however, many coupled systems leak energy as they metamorphose from one shape to another. Alireza Marandi, a member of the Kavli Nanoscience Institute at Caltech, wants to understand those leaky systems. To learn more about what he calls dissipatively coupled systems, he has developed an experimental platform that links together a network of optical resonators (cavities used to trap photons) and then studies how light leaking from the network affects the light that remains. His work could open the door to studying an entirely new set of technologically important materials systems.
Nanowires emulate the body’s cilia.
Cilia, the body’s microscopic hairs, do everything from clearing phlegm from our lungs to keeping cerebrospinal fluid flowing in our brain. Now, researchers led by Itai Cohen and doctoral student Wei Wang of the Kavli Institute at Cornell University have found a way to individually control approximately 1,000 nanostructured cilia. They can generate multiple streams of liquid and recombine them or create whirlpools or even 3D flows. The cilia array is based on a platinum-based actuator Cohen’s group developed to get robots to walk. As the voltage applied to 50-micrometer-long cilium rises and falls between -0.2 and +1 volt, the surface oxidizes and reduces. The associated expansion/contraction causes it to wave back and forth, pumping fluid at tens of microns per second. A typical cilia chip consists of 16 squares containing eight arrays of eight cilia each, or (16x8x8) 1,024 cilia. The device also has an electronic, solar powered clock circuit that controls the cilia without tethering it to a PC. This could allow technologists to eventually use the devices to run multiple diagnostics on a single drop of blood.
Congratulations
Omar Yaghi, co-director of the Kavli Energy NanoScience Institute at UC Berkeley, has been elected to the American Academy of Arts and Sciences for his work on metal-organic frameworks (MOFs), a family of porous crystals that have many potential applications.
Richard Wade-Martins, a member of the Kavli Institute for Nanoscience Discovery at Oxford University, and the school’s Department of Physiology, Anatomy and Genetics have received a $4.8 million grant from the Wellcome Trust to study the function of calcium in dopamine neurons in Parkinson's disease.
Katja Nowack, a member of the Kavli Institute at Cornell University, and Brad Ramshaw have received a New Frontier Grant from the College Arts and Sciences to develop a thermal microscope to image quantum states of matter that are invisible in conventional experiments.