Electrical Properties of Superconductor Altered By 'Stretching'
(Originally published by Cornell University)
May 26, 2016
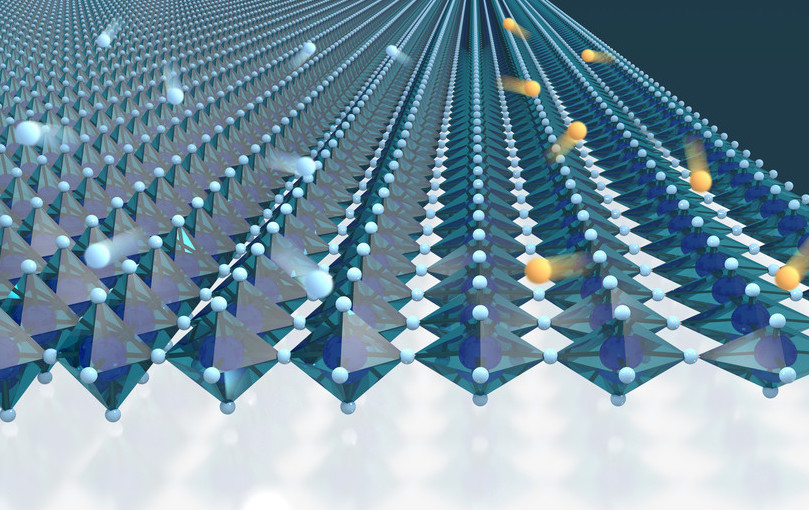
In the early 1970s, in the basement of Clark Hall, the Cornell team of professors David Lee and Robert Richardson, along with then-graduate student Douglas Osheroff, first observed superfluid helium-3. For that breakthrough, the catalyst for further research into low-temperature physics, the trio was awarded the 1996 Nobel Prize in physics.
Twenty years later, another Cornell-led team – working in that same building – has made an important discovery regarding the superconductor strontium ruthenate (Sr2RuO4,or SRO), often described as a crystalline analog of superfluid helium-3. What ties them together is the unusual way the electrons are paired together in SRO, and how the helium atoms are paired in the superfluid. That quality makes SRO intriguing for possible applications in quantum computation.
A team led by Kyle Shen, associate professor of physics, and Darrell Schlom, the Herbert Fisk Johnson Professor of Industrial Chemistry, both members of the Kavli Institute for Nanoscale Science at Cornell, has shown the ability to alter the electrical properties of the unique material through the application of strain – stretching thin films of SRO on top of a single-crystal substrate.
The group’s paper, “Strain Control of Fermiology and Many-Body Interactions in Two-Dimensional Ruthenates,” was published May 13 in Physical Review Letters. Collaborators included researchers from Stanford University, the University of St. Andrews, Scotland, and Max Planck institutes in Stuttgart and Dresden, Germany.
“You can pick different kinds of single crystals [of a substrate] where the atomic bond lengths are a little different, a little longer or shorter,” Shen said. “And when you deposit the SRO film on these crystals, they take on that length that was set by the substrate. And when you change these bond lengths in the SRO film, you can really dramatically alter its properties.”
The technique is used in silicon, to speed up the flow of current in a device. Shen and Schlom have published work on the use of “knobs” to tune, or control, material properties.
What the group was able to observe by photoemission spectroscopy and was able to graphically depict, was that the electrical charge carrier in the material switched from an electron (negative charge) to a hole (positive charge). An electron hole is the absence of an electron in a particular place in an atom.
“We had an idea that this might happen, but now you can really see it directly using photoemission,” Shen said. “You pull on the material, stretch it out, and when you do that, these charge carriers convert from one sign to another. And actually when you measure the flow of electricity in here, you find that, yes, one acts as if the charge carriers are electrons, and the other acts as if the charge carriers are holes.”
The challenge with superconducting is temperature. The record for high-temperature superconductivity is -94 degrees Fahrenheit; most superconductors work best at near absolute zero (0 degrees Kelvin, or -459.67 degrees). The transition temperature for SRO – the temperature at which it’s a superconductor – is around 1.5 degrees Kelvin.
There are still many challenges to overcome, but Shen is encouraged.
“It’s kind of a proof of principle, that we can see this change of the electrical behavior,” he said. “The next step is to boost up the superconducting transition temperature to a much higher temperature than 1.5 Kelvin, which would be amazing.”
Contributors to this work included current and former physics graduate students Bulat Burganov, John Harter and Daniel Shai; former postdoctoral researcher Carolina Adamo, Department of Materials Science and Engineering (now at Stanford); graduate student Andrew Mulder, School of Applied and Engineering Physics; former physics postdoctoral researchers Masaki Uchida and Phil King; and associate professor Craig Fennie, School of Applied and Engineering Physics.
This research was supported by grants from the Air Force Office of Scientific Research and the National Science Foundation, which supports the Cornell Center for Materials Research. Part of the work was done at the Cornell NanoScale Science and Technology Facility, which is also supported by the NSF.