From DNA to the Brain
by Lindsay Borthwick
How studying genetic information in our cells helps us understand the nervous system
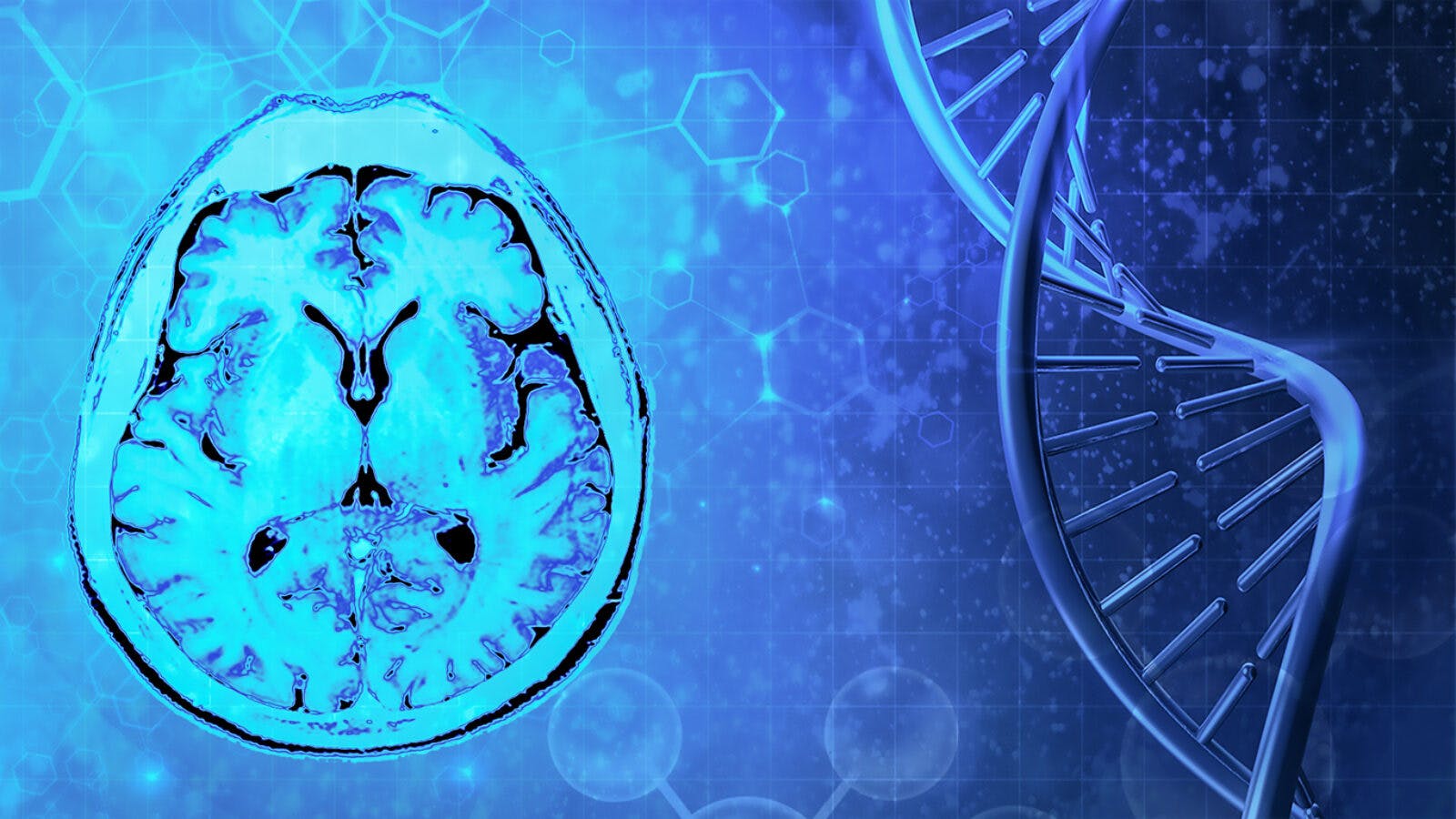
The Author
A series of recent studies from the Kavli Neuroscience Institutes illustrate how researchers are using powerful new tools for studying the genetic information in our cells to understand the nervous system. Plus, scroll down for a roundup of the neuroscientists whose personal journeys, ideas and discoveries have caught the attention of science storytellers on the Web.
All Vertebrates Great and Small
This year mark’s the 20th anniversary of the human genome. Since the publication of the first draft, the cost of sequencing has plummeted and the speed and accuracy of genome assembly have increased dramatically thanks to new techniques. The Vertebrate Genomes Project (VGP) is taking advantage of these powerful sequencing new methods to read the genetic code of all vertebrate species—more than 70,000. The goal is to generate a vast, open catalog of genomes to understand the evolutionary processes that shape these species and answer fundamental questions in biology. In April, the consortium published the first 25 high-quality vertebrate genomes, including a flagship paper, and the complete reference assembly of several bat species, the platypus, zebra finch—a songbird studied by neuroscientists seeking to understand language acquisition, and the common marmoset—a model organism in brain research. The VGP is led by Dr. Erich Jarvis, PhD, a member of the Kavli Neural Systems Institute at Rockefeller University, whose research focuses on unraveling vocal learning in songbirds and mammals.
Published in April in Nature, the VGP genomes yielded some surprises: a new assembly of the zebrafinch genome revealed eight new chromosomes and about 900 genes, including the regulatory region the birds’ need to learn a tune; and, in the marmoset, researchers found an unexpected amount of genetic diversity between maternal and paternal chromosomes, which has implications for their use as a model system.

Spotting Differences Between Neurons
A pair of studies out of the Salk Institute, led by researchers who are members of the Kavli Institute for Brain and Mind (KIBM) at Salk, used powerful new tools to sequence or tag genetic information in single neurons:
The first study introduces a new technique, termed Repair-seq, that uses synthetic nucleosides—the molecules that serve as building blocks for DNA—to identify “hotspots” in the genome in individual cells. These hotspots are damaged DNA regions. Unlike most other cell types, neurons rarely turn over, so DNA damage builds up over time. The new study showed that hotspots are not random, suggesting that neurons prioritize certain regions for repair, likely because they play a critical role in neuronal identity and function. “We’re excited about the potential of these findings to change the way we view many age-related diseases of the nervous system and potentially explore DNA repair as a therapeutic approach,” said co-corresponding author, Fred “Rusty” Gage, in a news article.

Another study used single-cell RNA sequencing (scRNAseq)—a technique that measures gene expression in individual cells—to distinguish subsets of spinal cord neurons in mice and determine their development origins. Researchers already have a classification system for spinal neurons, which relay messages from the spinal cord to the rest of the body, sometimes over long distances. But it lacks precision, especially for small subsets of cells. Getting a clearer picture of the different cell types in the spinal cord, by looking at their genetic signatures, could help researchers better understand movement, as well as spinal cord injuries and movement disorders, including neurodegenerative disorders like amyotrophic lateral sclerosis (ALS), at the cellular level. In the new analysis of more than 7,000 cells from mice, the team found genetic markers that distinguish long-range and short-range neurons and cut across existing classes of cells. Those markers will help the researchers study whether particular cell types are affected by injury or disease, and potentially how to grow healthy replacement cells. The research was led by Samuel Pfaff.
Eavesdropping on the Brain in Double
The brain is built of connections, and it is the crosstalk between interconnected neurons, circuits and regions that give rise to function. Now, researchers at the University of California, San Diego, have developed a device to monitor brain activity in various brain areas at once, and they have used it to study how two regions communicate during learning and memory. Duygu Kuzum, an electrical engineer, developed the brain implant, called Flexible, Insertable, and Transparent Microelectrodes (Neuro-FITM). In collaboration with Takaki Komiyama, she used the implant to record from neural populations in the mouse brain, specifically in the hippocampus and the dorsal cortex during sharp-wave ripples (SWRs). SWRs are bursts of synchronized neuronal activity that occur during sleep and rest that support both the consolidation and retrieval of memories. The team found that communication between the hippocampus and cerebral cortex is two-sided. In fact, “cortical activity often preceding hippocampal SWRs (in contrast to popular concepts that SWRs originate in the hippocampus and propagate to the cortex)," tweeted Komiyama. He is affiliated with the Kavli Institute for Brain and Mind.
Faces of Neuroscience
Researchers are using machine learning approaches to decode animal communication. But can they translate between species? “You can’t just have a Skype conversation,” commented Marcello Magnasco, physicist and dolphin researcher at Rockefeller University, where he is a member of the Kavli Institute for Neural Systems. “We will need to understand what it is to be a dolphin.” Read the full interview in The New Yorker.
In an episode of his podcast titled “Something in the Way You Move,” Alan Alda talks to neuroscientist Eman Azim, a member of KIBM at Salk, and his wife, neurosurgeon Sharona Ben-Haim, about how the brain controls movement.
Neuroscientist Nenad Sestan made headlines last year when his research team restored some neural function in the brain’s of pigs that had been dead for a few hours. But his contributions to our understanding of the development of the cerebral cortex are much deeper than that one experiment. A new profile of Sestan describes his journey from rural Croatia to medical school to leading a research lab at Yale—”the Mecca if you wanted to study the cerebral cortex,” where he became an assistant professor in 2002. Sestan is a member of the Kavli Institute for Neuroscience at Yale.
A new video from researchers at the Kavli Institute for Systems Neuroscience in Trondheim, Norway, spotlights their key advances in 2020.