From Movies of Catalysts to Carbon in Soil
by Alan S. Brown
Research highlights from Kavli Nanoscience Institutes
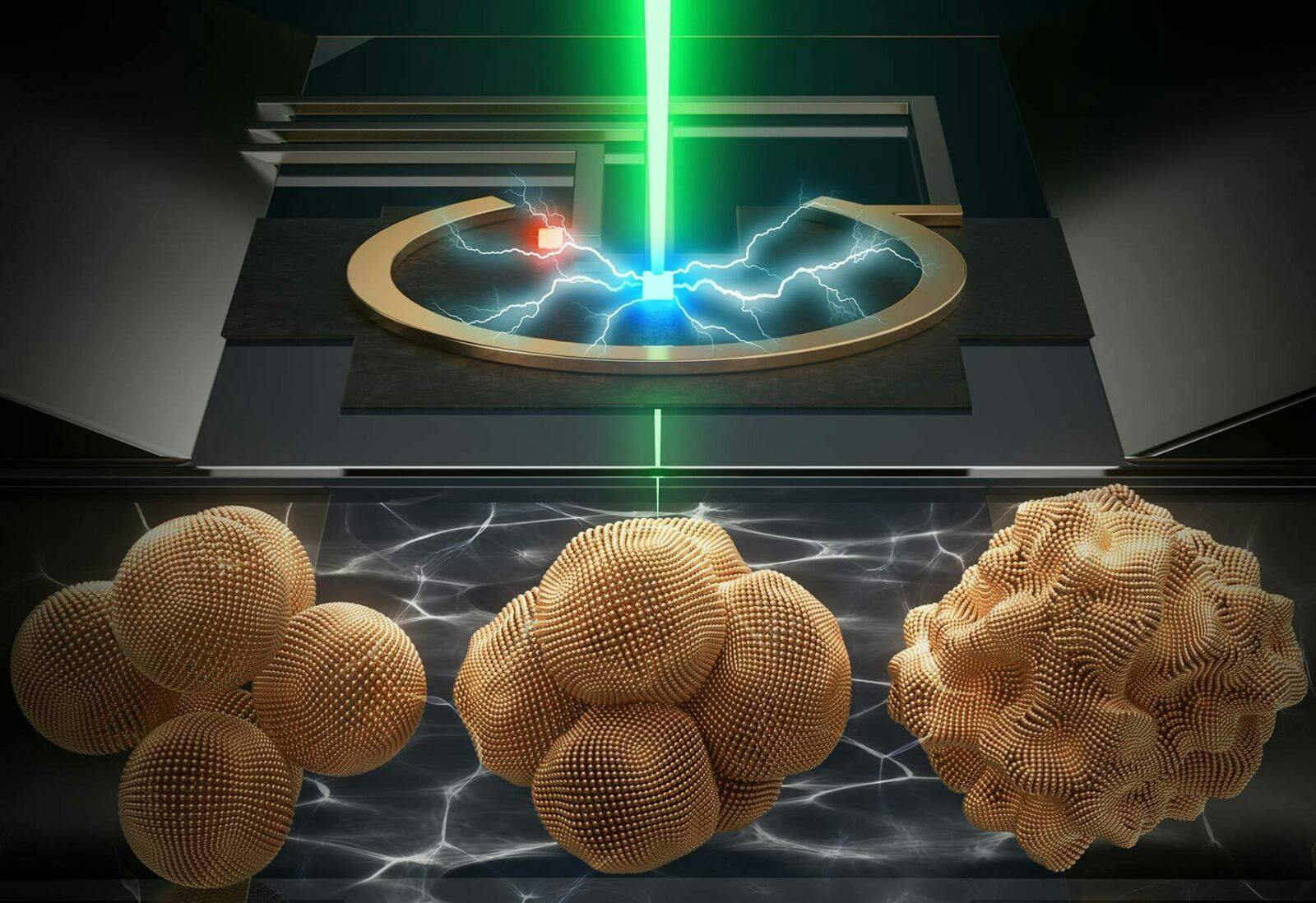
The Author
In this collection of Kavli Institute Nanoscience highlights, researchers created real-time movies to show the mechanism of copper catalysts at work; found a way to boost the properties of 2D superconductors; used crowd sourcing to rapidly train AI models of antibacterial resistance; discovered that inherent defects can reveal universal properties of the metal-insulator transition with possible application to quantum spin liquids; and studied a relationship between soil microbes and soil carbon storage.
Real-time movies show catalyst at work.
Copper is the only catalyst known to transform atmospheric carbon dioxide into long-carbon-chain molecules for fuel and chemicals. However, more understanding is required to reliably produce desirable products with higher yields. Now, Yao Yang, a postdoctoral researcher in the lab of Peidong Yang, co-director of the Kavli Energy NanoScience Institute at Berkeley, has made a key breakthrough. Prior to coming to Berkeley, Yang studied with Kavli Institute at Cornell co-director David Muller, who developed an electron microscopy technique that enables him to examine individual atoms. In a collaboration between the two Kavli Institutes, Yang invented a specialized liquid cell that enabled him to use the electron microscope to image how copper catalyzes CO2 to form longer carbon chains. These movies show where the catalyst is most active and offer insights into ways to rationally engineer them to improve their specificity. This is of critical interest to Peidong Yang’s group in particular, where researchers are developing ways to use solar energy to drive CO2 reactions.
Graphene sandwich boosts superconductivity.
Five years ago, MIT researchers found they could turn graphene into a superconductor by offsetting two atomically thin (2D) layers of graphene by 1.05 degrees, called “the magic angle” for obvious reasons. They also discovered that they could switch this twisted graphene stack between a superconductor and insulator by applying an electrical field. One year later, they replicated these properties using two layers of untwisted graphene. The resulting stack is much easier to make, but also more delicate, harder to tune, and works only at two orders of magnitude lower temperatures. Now, Stevan Nadj-Perge at Kavli Nanoscience Institute at Caltech has found a way to combine the properties of both materials. The trick is to cap untwisted graphene with an atomically thin layer of tungsten diselenide, another 2D material with interesting electrical and optical properties. The resulting sandwich turns superconducting at 10 times higher temperatures than untwisted layers and is easier to fabricate. It also has fewer defects and better tunability, making it a good material for studying superconductivity and perhaps commercializing superconducting devices.
Crowd-sourcing infection inspection
Antibiotic-resistant bacteria kill more than 700,000 people annually and this could grow to 10 million by 2050. Why? One reason is that it takes a day or more to diagnose a severe infection, so clinicians treat patients with broad spectrum antibiotics. This shotgun approach increases the amount of antibiotic-resistant bacteria in circulation. One way to circumvent this problem is to develop faster diagnostic tests. Achillefs Kapanidis, a member of the Kavli Institute of Nanoscience Discovery at Oxford University, wants to apply machine learning models to analyze test results faster and more accurately to determine which antibiotics would attack the pathogen. First, though, Kapanidis needs a database to train his model. He already has thousands of microscope images of bacteria exposed to antibiotics, but needs to annotate them so the model can learn faster. So he turned to the website zooniverse.org to line up more than 1,500 volunteers to examine the images for changes in bacterial cell structure, which indicate whether bacteria are antibiotic resistant or sensitive. He hopes crowd sourcing will speed development of his machine learning model and lead to faster infection testing.
Could moiré lattices produce quantum spin liquids?
In 2021, two Kavli Institute at Cornell (KIC) researchers, Kin Fai Mak and Jie Shan, demonstrated they could use an electrical current to switch a moiré lattice from conductor to insulator and back again. A theory published in 2008 predicted this and other key aspects of the material’s behaviormetal-to-insulator transition. It also predicted that the lattice’s electrical resistance would jump by a “universal” n amount governed by fundamental constants of nature but that did not appear to happen. As KIC member Debanjan Chowdhury set out to discover why not, . Instead of looking at the theory, he focused on imperfections in the lattice, which counterintuitively do help reveal the universal features of the transition. He found that defects within the lattice created “islands” of three types: insulators, conductors, and conductors with a high levels of resistance governed by fundamental constants, as predicted. Electrons had to find a way through these islands. His findings not only confirm the theory but also suggest moiré lattices could act as quantum spin liquids, materials where an electron’s charge is freed from its spin. The result could be exotic quantum states that could encode information in nonlocal excitations and possibly a new direction in quantum computing.
Microbe death governs carbon in soil.
The Earth’s soil stores more carbon than its plants and atmosphere put together. Kavli Institute at Cornell’s Johannes Lehmann has teamed with Tessa Camenzind of Freie Universität Berlin, where he is a visiting researcher, to examine how natural microbe death changes the quality of soil and its ability to retain carbon. Microbes, it turns out, die in many ways. They compete with other organisms for food and territory while facing drought, extreme heat, freezing temperatures, and human activity. Bacteria and other single-cell organisms prey upon them; viruses infect them. How an organism dies— whether from dehydration, digestion by a bacteria, or viral reprogramming—determines how much carbon it will sequester and for how long. Soil microbiomes are incredibly complex, but this is an important contribution to our understanding of how soils store and manage carbon.