Frontiers in Astrophysics
How did the universe begin? When—or for that matter will—it end? Answering questions about our very existence is often at the heart of astrophysics.
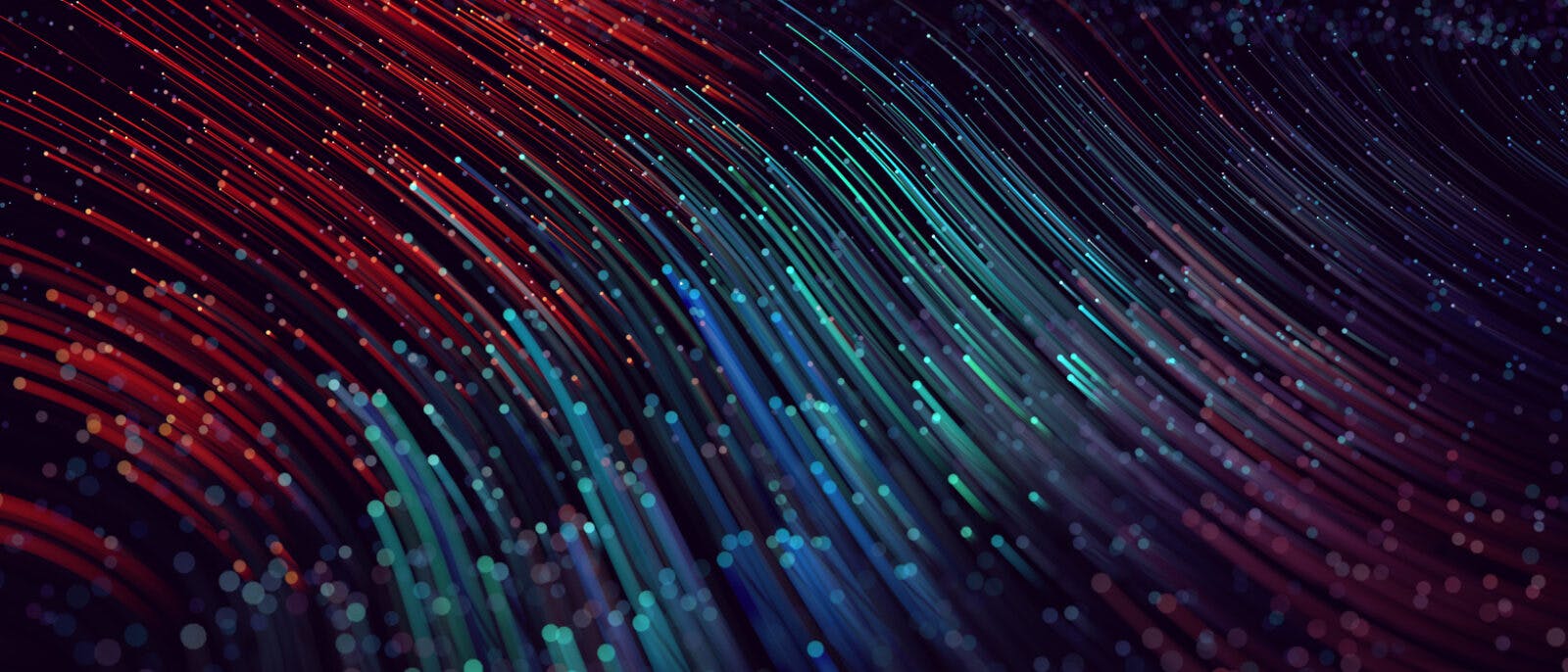
The Researchers
Only in the last century did scientists really begin to understand the physics of the cosmos.
Putting eyes to telescopes and pencils to paper, 20th century observers and theorists were the first to grasp the vastness of space and glimpse the diversity of its contents. The points of light rotating overhead at night, those explorers discovered, are to the cosmos as a cover to a book. And the book turned out to be an elaborate mystery story; full of peculiar characters and surprising plot twists, with the ending still unwritten.
Today’s scholars of the nighttime sky tell a story of a universe incomprehensibly huge. Once misjudged to be a fixed sphere of stars encircling the sun and planets, the cosmos is now a ballooning expanse of space populated by billions and billions of galaxies, each containing billions and billions of stars. The galaxies aggregate in intricate clusters, forming great walls that envelop vast voids. Light from the most distant galaxies reaches Earth only after a transit time of billions of years.
And day after day, the universe grows bigger. Galactic clusters recede from one another at rapid speeds as the space separating them expands. Permeating all the intervening space is a faint glow of cosmic radiation, the apparent remnant heat from a cataclysmic explosion – the Big Bang – that burst the universe into existence almost 14 billion years ago.
So much is known; much more is not.
Today’s astronomical explorers seek answers to several deep questions about the nature of space, the astrophysical objects it contains, and the universe’s composition, history and future. Guiding this quest are the 20th century’s grandest theoretical accomplishments: quantum mechanics and Einstein’s theory of general relativity. Quantum theory specifies the ground rules for explaining matter’s basic particles and the forces acting between them, while general relativity governs the large-scale behavior of the cosmos, describing gravity, space and time.
Despite their great success in realms small and large, quantum theory and general relativity have left scientists in the dark about several fundamental issues.

Figure 1. Timeline of the Universe illustrating the expansion of the universe following the big bang and subsequent formation of atoms, stars, galaxies and clusters of galaxies. (Courtesy of Bryan Christie Design; Scientific American, February 2004, p.47)
The Search for Answers in a Dark Universe
For one thing, astronomers cannot say what the universe is mostly made of. Add up all its mass and energy, and the stars and gases built from ordinary matter account for a mere four percent of it. Almost 25 percent or so seems to be some form of matter, but too dark to be directly viewed, and not of any sort ever found on Earth. The rest – 70 percent of the cosmos or more – masquerades as an invisible (or “dark”) energy field, exerting a repulsive force on space itself.
“Dark energy has the remarkable feature of having negative pressure,” says Rocky Kolb, of the Kavli Institute for Cosmological Physics at the University of Chicago. “This drives the universe to accelerate – to expand with an ever increasing expansion velocity.”
Identifying the true nature of dark energy and dark matter is the prime directive guiding the pursuits of 21st-century astrophysicists and cosmologists. But besides dark energy, space contains a menagerie of other exotic objects and phenomena taxing the human mind’s explanatory powers.
Some stars explode, for instance, brightly enough to momentarily outshine an entire galaxy. Such explosions, called supernovae, underlie many astrophysical mysteries. Many such explosions leave behind dense cinders called neutron stars, packing a mass of several suns into a ball the width of a small city. Neutron stars often beam radio signals through space as they rotate, like a lighthouse, enabling earthbound astronomers to record data for testing their theories.

Figure 2. This computer-generated image shows the simulated distribution of dark matter in a galaxy cluster formed in the universe with dark energy. The clumps are locations where galaxies form. (Courtesy of Andrey Kravtsov)
After a very massive star blows itself up, the remnant may be denser than a neutron star – so dense, in fact, that it collapses under its own mass, crushing itself into nothingness and leaving behind its gravity. The result is a black hole – a cosmic sinkhole with gravity concentrated so strongly that any object entering within its boundary remains forever trapped. On its journey into black hole oblivion, however, matter heats up and emits streams of radiation that signal the black hole’s presence to the outside world.
Black holes, neutron stars and all other known forms of matter cannot account for what astronomers see through their telescopes. Galaxies spin with speeds exceeding the limits implied by their mass. And galaxy clusters hang together more closely than possible unless some invisible glue binds them together. An additional source of gravity, matter unseen (and therefore called dark) must exist to hold the universe together, so to speak, and sculpt its majestic structure.
But what could that dark matter be? Physicists who understand atoms, and the particles from which atoms are made, say known forms of matter could not supply the galactic glue. Limits on the density of ordinary matter can be calculated based on the way atoms were cooked up in the big bang from a hot primordial soup of quarks and electrons. Such calculations show that matter made from particles familiar on Earth makes up a small fraction of the matter in space.
Physicists theorize, however, that other sorts of particles exist, perhaps “superparticles,” much more massive than those making Earthly matter. In several underground laboratories around the world, physicists attempt to capture such particles as they stream through space – so far without success, but still with hope.
Efforts to detect the presence of superparticles in the cosmos has led to the development of a new field of study called particle astrophysics. “There’s a growing recognition now in the whole community that particle astrophysics is a legitimate part of particle physics,” says Josh Frieman, also of the Kavli Institute for Cosmological Physics in Chicago.
That new field has forged close ties between astrophysicists and physicists working at particle colliders, where known bits of matter are smashed together in hopes of producing particles never before seen. If they can’t be caught in flight through space, perhaps dark matter particles can be created in colliders. And producing the particles in colliders would offer additional benefits.
“Everybody hopes that they’ll detect dark matter directly in some underground detector,” says Frieman, “but to really learn about what that particle is, we need to see it produced in a collider so we can really probe its properties.”
Einstein Revisited
Even more perplexing than dark matter is dark energy. Einstein anticipated something like it, adding a term to his equations that describes a constant energy residing in the vacuum of space, everywhere of equal strength at all times.
Einstein abandoned that idea, but modern cosmologists have revived it, in light of compelling evidence that the universe expands at an accelerating rate. In fact, quantum theory suggests that such a “vacuum energy” should indeed be present, everywhere in space. But calculations predict a density vastly exceeding the observed amount. In fact, the amount of vacuum energy predicted by theory would blast the universe apart so fast that no structure would form – meaning no stars, no planets and no people.
Obviously that prediction is wrong. Consequently some physicists question whether dark energy really exists, suggesting that the accelerating expansion of space reflects large-scale deviations from the law of gravity. If so, Einstein’s general theory of relativity needs to be modified. Others believe the dark energy is a cosmic fluid that alters its strength over time, unlike the constant vacuum energy foreseen by Einstein.

Figure 3. Supersymmetry theories postulate that the two types of particles found in our universe (bosons and fermions) cannot exists withour each other. According to supersymmetry, for each type of fermion which exists, there must also exist a boson with many of the same properties - known as its superpartner. In many supersymmetric theories, the ligh test superpartner particles is stable and weakly interacting, making it a good candidate for the dark matter of our universe. (Courtesy of Dan Hooper)
Observers continue to seek more data from space that might resolve the dark energy mystery. Meanwhile, theorists seek guidance from the mathematics of general relativity and quantum mechanics. But these two pillars of physics, the 20th century’s greatest theoretical achievements, seem fundamentally incompatible. Many experts believe that understanding dark energy must await a theory that unifies quantum physics with Einstein’s gravity, tying up the remaining loose ends in the cosmic story.
One leading candidate, known as string theory, has been thoroughly explored for the past two decades, so far without ultimate success. But many experts believe string theory’s mathematical power, once fully understood, will be great enough to conquer all the problems of the cosmos.
“There is very much a sense that we are discovering something, that there is some structure out there which unites gravity and quantum mechanics in some unique way, and we haven’t yet discerned its full form,” says string theorist Joe Polchinski, of the Kavli Institute for Theoretical Physics at the University of California, Santa Barbara.
String theory conceives matter’s basic particles to be tiny bits of vibrating string, called superstrings. Strings vibrating in different modes represent different basic particles, sort of like the way a violin string can produce various musical notes. String theory’s math makes sense, though, only if space possesses more than three dimensions – perhaps as many ten. Such extra dimensions go unnoticed, string theorists have proposed, because they are so small, on the size scale of the strings themselves. And a string is smaller than an atom as an atom is smaller than the solar system.
Are There Countless Universes?
While string theory was originally explored in the realm of particle physics, theorists have more recently studied its implications for cosmology. Its most astounding cosmological consequences stem from the possibility that the extra dimensions of space might be bigger than previously believed. Large dimensions would remain invisible because matter and light are stuck like glue to 3-D space. Gravity, however, would be free to travel through other dimensions. If large dimensions actually exist, the visible universe might be just one of many 3-dimensional bubbles (or “branes,” short for membranes) floating in a higher dimensional space. If so, new possibilities emerge for explaining dark matter and dark energy.
Sometimes advertised as a possible “theory of everything,” string theory might provide a recipe for all the basic properties of nature, including a precise specification of how dense the dark matter should be. But some theorists believe that string theory will tell a different story – that in fact, the amount of dark energy in the cosmos cannot be precisely specified by any theory. In fact, some versions of string math suggest, dark energy could exist in any possible amount, taking on different values in different realms of reality. These “realms” might even be parallel universes, independent bubbles of space blown into existence by other big bangs.
Perhaps, this view implies, the universe that humans inhabit is only one among countless others, each with a different dark-energy density. Humans find the amount of dark energy in this universe to be small because that’s the amount that is compatible with life existing in the first place.
Such reasoning relies on what is known as the anthropic principle, the idea that the properties of the universe must be hospitable to life, because otherwise we wouldn’t be around to discuss it. Anthropic reasoning is bitterly opposed by many physicists who regard it as giving up on the grand goal of finding a final theory that specifies the properties of the universe in every detail. But others say permissible solutions for cosmic mysteries must not be prejudged, and solving the deep problems of the cosmos may require radical revisions in the way scientists think.
In that sense the situation sounds similar to that of a century ago, when astronomers realized that the Milky Way galaxy, home to sun and Earth, was not the entire universe, but only one of billions of “island universes” or separate galaxies. Today the prospect exists that the known universe is only one of many others, too, and the very definition of “universe” needs rethinking.
Whether such a profound rewriting of the book of the cosmos is in order will await the outcome of further explorations by today’s astrophysicists and cosmologists. In any case, resolving today’s paradoxes will no doubt produce profound scientific advances, just as quantum mechanics and general relativity emerged from efforts to solve the mysteries of a century ago.
“We’re faced with tremendous questions, and often real advances come when you’re faced with big problems,” says Kolb. “And I think the problems that we’re faced with now, as we begin the 21st century, are as fundamental as the problems that we faced in the 20th century that led to quantum mechanics and general relativity.”
back to top