Are Computers Finally Going Quantum?
by Melissae Fellet
As the race to build the world’s first quantum computer heats up, tech industry heavyweights like Microsoft, Intel and Google are investing in research.
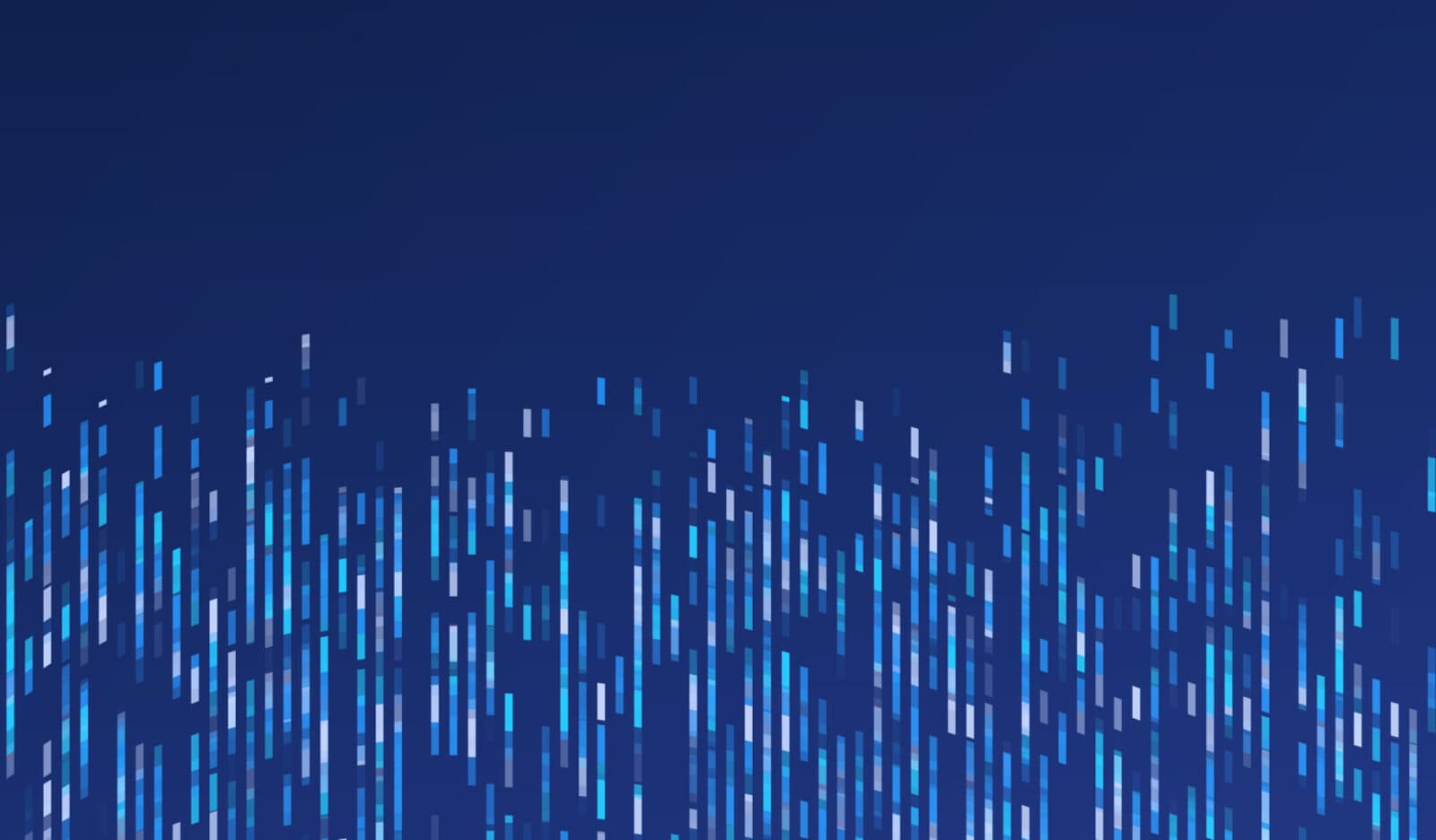
The Author
The Researchers
If one of these teams of physicists and engineers wins the race to build the world’s first quantum computer, it would out-compute classical computers by a long shot and help tackle currently unsolvable problems in fields ranging from finance to cybersecurity, and from drug development to quantum physics itself.
But there are still major technical challenges to overcome, including how to scale up current designs to link hundreds or thousands of quantum bits (qubits), the basic information carriers in a quantum computer, and how to control qubits’ strange behavior. The Kavli Foundation spoke with three quantum researchers about recent advances in the field, the challenges of building quantum chips, the potential of a quantum future—and how soon we’ll get there.
The participants were:
- LIEVEN VANDERSYPEN - is a physicist at Delft University of Technology, co-director of the Kavli Institute for Nanoscience at Delft, and a member of QuTech, a research center that is developing quantum technology, in partnership with Intel and Microsoft.
- SHOHINI GHOSE - is a physicist at Wilfrid Laurier University, where she studies how the theory of quantum physics could change computing and communication.
- JOHN MARTINIS - is a physicist at the University of California, Santa Barbara. He is working with Google on the development of a quantum computer using superconductive materials.
The following is an edited transcript of their roundtable discussion. The participants have been provided the opportunity to amend or edit their remarks.
THE KAVLI FOUNDATION: Why are tech companies racing now to build a quantum computer?
JOHN MARTINIS: Google has giant datacenters to crunch data, but they’re hitting the limits of computing power. They think it’s time to look at alternate technologies to overcome those limits, and quantum computing is part of that mix. The idea that we could have exponentially powerful processing power with a quantum computer is very appealing.

LIEVEN VANDERSYPEN: I think companies see the opportunity to contribute to a new technology that can have a really tremendous impact by solving problems that are currently out of reach. Quantum computers work fundamentally differently than conventional computers, which use transistors to transmit bits of information as electrical pulses encoded numerically as a 0 or a 1. To double the computing power, you double the number of transistors.
In quantum computers, individual atoms, electrons or particles of light carry information in quantum bits, or qubits. Qubits can exist not only in 0 or 1, but also in any blend of 0 and 1. When two qubits are in a blended state together, they also share information with each other. This blending and information sharing means a quantum computer doubles in power for every quantum bit that you add.
TKF: Picking up on that, what kinds of problems might quantum computers be able to solve?
SHOHINI GHOSE: Quantum computers have a definite advantage for searching through unsorted information, which may be why one of the big companies interested in this is Google. With a quantum search, we can search through hundreds of billions of possibilities as quickly as a classical computer can search through a million possibilities. Quantum computers could also factor very large numbers, which would enable them to break current encryption methods. Yet we could still send secret messages even if we had a quantum computer, because quantum physics enables us to design encryption protocols that would let us know if a message has been intercepted.
MARTINIS: Google also uses tremendous amounts of processing power for algorithms used widely in their language translation, voice-recognition and image-recognition software. Computers use these algorithms to categorize a large set of different images, for example. Then when the computer receives an image it’s never seen before, it can identify it using the categories it previously learned. A quantum computer would use less processing power for these algorithms because it could quickly jump between categories and scan multiple categories at the same time.
VANDERSYPEN: A quantum computer will also be valuable for solving the types of problems that even today’s most powerful computers cannot handle. The ones that most motivate me are simulating the properties of molecules and materials. That may sound a bit narrow, but I’m told that half of the time available on public supercomputers today is spent solving problems in chemistry and materials science. If you can do that more efficiently, it can help us to more efficiently design new drugs and develop new materials.
TKF: How is the tech industry’s increased interest in quantum computing helping your research?

MARTINIS: As you start to scale up from one quantum bit to many, the engineering problems get very challenging. I think that is where people in industry—in our case at Google—can really help out. Physicists have made a lot of progress in understanding how to build quantum systems, and to me it seems like the right time to put in a lot of engineering effort along with the physics.
VANDERSYPEN: It’s similar for us. Our research center, QuTech, began to work closely with Intel a year and a half ago. We’re focusing on the fabrication of large numbers of quantum bits to ensure that they all work and work well without too many personality quirks. Reliably manufacturing quantum bits has been one of the key obstacles to moving forward.
At the university, we have a state-of-the-art clean room where we make quantum chips, but it’s optimized to produce chips for the creative science that is typical in academia, rather than for highly repeatable manufacturing like you would see in the semiconductor industry. Working with colleagues at Intel and having access to their clean-room facilities is going to boost to how fast we can move.
TKF: What else makes it so challenging to build a quantum computer?
VANDERSYPEN: There are many components on a computer chip, and it’s not easy to design qubits so their quantum behavior can actually be observed and used. Fluctuations from all the stuff around the qubits will randomly change the qubits so that we can no longer use them for a quantum computation.
Over the years, researchers have learned to maximize the amount of time quantum bits remain in quantum states. We call that time the “coherence time,” and it has gone up by orders of magnitudes for several technologies, including the qubits that John works on and the ones that I work on.
MARTINIS: There’s a fundamental tradeoff inherent in quantum computing: interactions between multiple qubits increase a quantum computer’s power, but those interactions also cause the qubits to lose their quantum states. Once this happens, the qubits act just like bits of information in a classical computer. As people try to build these systems, the new challenge is going to be getting everything to work at the same time.
GHOSE: As a theorist, I’m not building quantum computers. But one of the challenges we face is figuring out how to solve problems efficiently in a quantum way, so that we can predict which property of a quantum computer would help solve a particular problem. We understand a little bit about why certain quantum algorithms work better than others, but we don’t understand the general reason why a quantum algorithm works well in one situation and not another.
"Physicists have made a lot of progress in understanding how to build quantum systems, and to me it seems like the right time to put in a lot of engineering effort along with the physics."—John Martinis
TKF: John and Lieven, you are both making qubits using different materials and quantum particles, while also leveraging existing engineering knowledge and infrastructure. What are some specific advantages of your different approaches?
VANDERSYPEN: In the qubits that I work on, the amount of time quantum bits remain in quantum states is several orders of magnitude beyond qubits like the ones John works on. That time gives you a lot of flexibility in how to design circuits. We don’t need to be as worried about doing everything quickly.
MARTINIS: Our big advantage is that we’ve been getting quantum chips to work very well for quite a few years. We’ve done experiments with nine qubits that work well, and we’re working on a 50-qubit chip for testing this year. We also know that our devices have low error rates. So our approach seems scalable and workable.
TKF: How does the computing power of a 50-qubit quantum computer compare to a classical computer?
MARTINIS: It’s close to the typical memory of a supercomputer. We want to run a simple algorithm on our 50-qubit chip and compare the results with those produced by the biggest supercomputers. We're not actually going to solve a useful problem. We're going to show that our quantum chip can do powerful computations that can only be checked with the biggest supercomputer.
We’re hoping that this kind of experiment will impress the executives in Silicon Valley by showing that quantum computers are not just some way-out physics idea. Our experiment, which we hope to test this year, is also going to demonstrate that an actual quantum computer is operating properly at this scale. Frankly, the physics has not been investigated on this size scale before.
"I think the development of a quantum computer is a gradual process of learning how to control and master increasing numbers of qubits [quantum bits]. There isn’t going to be a single point at which we have a quantum computer and the previous day we didn’t."—Lieven Vandersypen
TKF: Shohini, can you give us an idea of what happens when you have a large number of qubits interacting on a chip?
GHOSE: We know that as you add more and more qubits, they get connected in this thing called entanglement. They literally start losing a bit of their identities because their connection becomes so powerful that all the information they carry becomes part of a joint system.
But we don’t know a lot about large-scale entanglement. In my research, I try to understand entanglement by looking at what it takes to generate it, classify different types of entanglement and identify tasks where particular entanglement types could be useful. It’s a difficult problem because every time you add a qubit to the analysis, the size of the math problem doubles. But when we do explore it, we find surprises all the time. There’s still so much to learn about how quantum particles connect to each other.
TKF: Does that mean we will need a quantum computer to explain how a quantum computer works?

GHOSE: Currently, we do our research using classical computers to simulate a relatively simple quantum system. But if you go from three to four to five qubits to 10 qubits, the system will be too complex to simulate this way.
One of the things that a lot of people are excited about is quantum simulators, where you use a quantum computer to simulate the quantum physics of another system. A quantum computer would have the power to run this type of simulation. But then the question becomes: How do you program a quantum computer to do a quantum simulation? That’s something that a lot of people are working on. If I had time, I would work on it too because it would be a powerful tool.
TKF: So how close are we to having the first quantum computers that could solve any problem like our current computers can?
GHOSE: In a sense, we already have basic quantum computers because researchers are working on a variety of ways to transmit and process information using quantum bits. In the near term, I think industry will focus on building a quantum computer that does one task really well, but it may not be a universal quantum computer that can perform any calculation in the way that conventional computers can.
"There’s still so much to learn about how quantum particles connect to each other."
—Shohini Ghose
MARTINIS: Many people have claimed to have built very small quantum processors. If you want to define a quantum computer on the basis that it can do something useful, then that’s not probably going to happen in 2017. But we’re definitely moving in that direction. We hope to show that a quantum computer can compute something powerful this year. It’s kind of a stretch goal for us, but we think we have all the technology.
VANDERSYPEN: I think the development of a quantum computer is a gradual process of learning how to control and master increasing numbers of qubits. There isn’t going to be a single point at which we have a quantum computer and the previous day we didn’t.
It is a very exciting time! And it’s such a very different feeling from some years ago when there was a lot of academic curiosity and interest in quantum computing but also a lot of skepticism. Now there’s very much the sense that this could be for real. I think the participation and contribution of industry is really going to advance our field, and that will be very exciting to watch.
Sidebar: What is a quantum computer?

Classical computers transmit bits of information as electrical pulses encoded numerically as a 0 or a 1. In quantum computers, individual atoms, electrons, or particles of light carry information in quantum bits, or qubits. Following principles of quantum mechanics, these particles can exist in 0, 1, or various probabilities of being either 0 or 1, called superposition. With qubits in superposition and communicating with each other through a property called entanglement, quantum computers can solve a problem by examining multiple solutions simultaneously. The result is extra computing power and speed.
But keeping the "quantumness" in qubits is a big challenge. Multiple qubits need to interact with each other to solve problems in a quantum computer, but any interaction with other particles causes them to lose their quantum states. Once a qubit settles into either a 0 or 1, it acts just like a bit in a classical computer.
As the result of research over the past decade, scientists can now build qubits that remain connected 10,000 times longer than before. But still less than 10 qubits can be fully quantum-ly connected.
In January 2017, the company D-Wave released a quantum computer containing 2,000 qubits. But few of those qubits interact with each other to their full quantum potential. While some quantum behavior may happen on D-Wave’s chip, it’s not providing much extra computing power: the D-Wave computer can solve one problem about as efficiently as a laptop, says John Martinis, at University of California, Santa Barbara.
Engineers built the D-Wave quantum computer specifically to solve a particular problem more efficiently. Martinis, Lieven Vandersypen at TU Delft, and other quantum computer researchers have a different goal: To build a universal quantum computer (see infographic) that could run any quantum algorithm the way our current computers run any standard program. Because those chips utilize all the various types of quantum behavior, the researchers will be able to use all the computational power that Nature will allow, Vandersypen says.