Galactic Mysteries Abound
by Adam Hadhazy
While ultra-numerous and there for the taking observationally, galaxies have so far resisted attempts to squarely and comprehensively describe their origins and behaviors
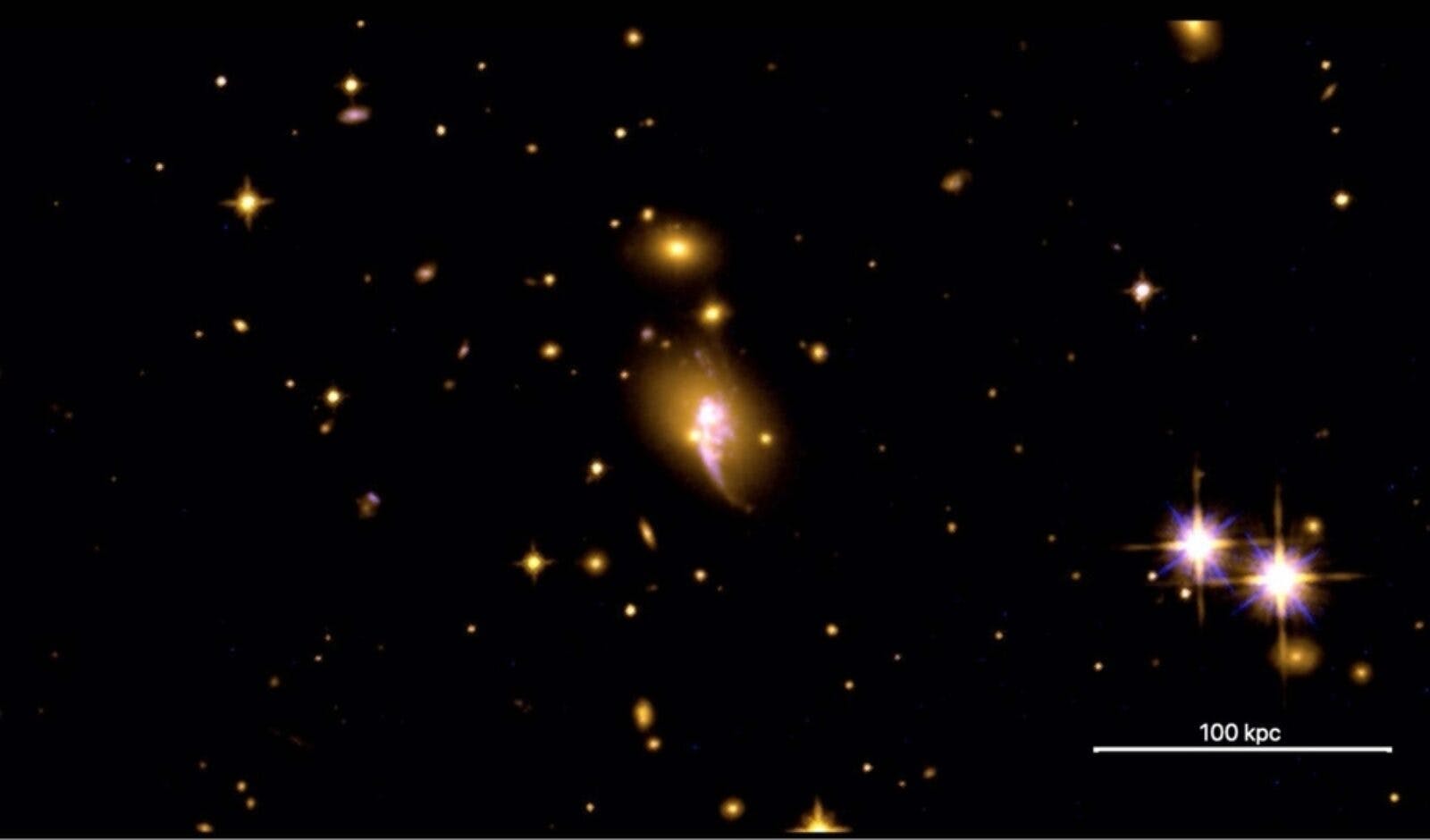
The Author
Depending upon whose estimate you believe, the observable universe contains somewhere in the vicinity of 200 billion to perhaps two trillion galaxies. The so-called Local Group, the collection of galaxies our Milky Way gravitationally associates with most closely, has at least 80 galactic members. Yet belying this abundance and decades of intensive study, there's still an awful lot we don’t quite get about galaxies. How exactly they formed and have changed over the universe's 13.8-billion-year history is still very much a matter of brow-furrowing consternation. We can fathom that galaxies took some time to form after the Big Bang, though whether it was a billion years or even as little as several hundred million remains to be sorted out. Whether galaxies formed in association with the supermassive black holes found in the cores of so many of them, or if the black holes came first or after the fact compared to the budding galaxies themselves, is likewise ill-understood. We have plenty of observations pointing to a hierarchical buildup and organization of galaxies over time, with galaxies starting off puny and misshapen and then, through mergers with each other, growing into the structured behemoths that become more common in the modern cosmos. But an increasing number of glaring exceptions to this galactic evolution pipeline threaten the entire scheme. Some recent studies involving Kavli astrophysics institutes-affiliated scientists have added to these consuming mysteries. It seems that patience will be key to solving the riddles that galaxies pose—that, plus a great deal of scientific grit. The galaxies are right there under our noses, oblivious to our intentions, but entirely amenable to our curiosity.
Some galaxy clusters masquerade as single galaxies, survey finds
As its name implies, the Clusters Hiding in Plain Sight (CHiPS) survey has sought out galaxy clusters overlooked despite decades of cosmic scouring. Led by researchers at the Massachusetts Institute of Technology’s Kavli Institute for Astrophysics and Space Research, the six-year effort recently presented its cumulative results. Of note, CHiPS has turned up three "new" galaxy clusters long mistaken for being a single galaxy when viewed in x-ray light. One new cluster is similar to the famous Phoenix cluster discovered in 2012. Both represent rare instances where galaxy clusters can be dominated by a lone, ultra-bright, star-forming galaxy that overwhelms the whole assemblage in telescopic observations. The newfound cluster also has a strange, twisted shape, suggesting it’s the product of two clusters merging. The overall results suggest that 1% of galaxy clusters have been missed in x-ray observations—a small, but not insignificant portion of the total that must be accounted for with high accuracy in order to hone cosmic structure and galaxy formation models.
Highest-energy galactic cosmic ray source revealed
Roger Blandford of the Kavli Institute for Particle Astrophysics and Cosmology is part of a team that has traced the highest-energy cosmic rays in the galaxy to a star-forming region. The result strongly suggests that supernova remnants, such as neutron stars and black holes, are not the sources of the rays, as previously suspected. Instead, the source appears to be environments where massive stars form and where high-energy particles can be accelerated to even higher energies. Those particles eventually reach Earth and smash into the atmosphere with gusto. The findings will help researchers better understand the origins and generation of certain cosmic rays.
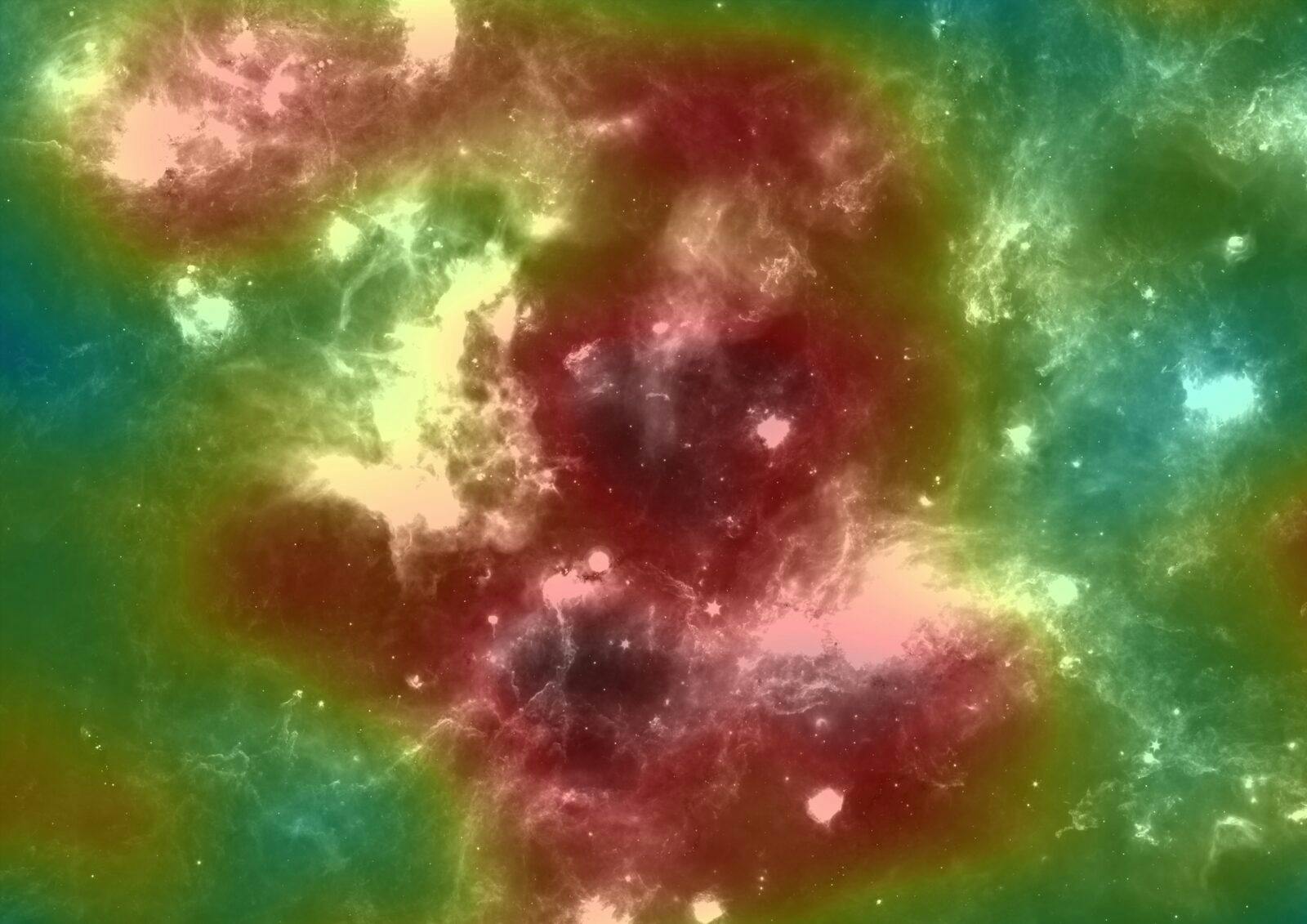
Probing the nature of dark matter with the tiniest, dimmest galaxies
To get a better bead on dark matter, the mysterious substance thought to outnumber normal matter some five to six times over, astrophysicists are studying the smallest, faintest galaxies in the local universe. These galaxies have very few stars, which translates to very few supernova explosions that theoretically could scatter dark matter. Those conditions make the ultrafaint dwarf galaxies ideal laboratories for testing out the self-interacting dark matter (SIDM) theory. According to this theory, dark matter particles can influence each other and prevent an overdense buildup of dark matter in galactic cores. Members of the Kavli Institute for the Physics and Mathematics of the Universe (Kavli IPMU) studied the motions of stars in 23 ultrafaint dwarf galaxies to put the theory to the test, based on how dark matter interacts with matter (composing the stars) via gravity, thus revealing galactic dark matter distribution. Overall, the findings point to dark matter being quite dense in galactic cores, undercutting the SIDM theory.
Stunningly advanced and stable galaxy in the fledgling universe
The conventional theory of galaxy evolution holds that galaxies started out small and in chaotic states, eventually merging over the eons and developing more organized structures, such as spiral arms, stable disks, and central, star-filled bulges. Discoveries of remarkably precocious galaxies, however, have challenged this framework. Now a new study led by Kavli Institute of Cosmology, Cambridge researchers has really thrown a wrench in the works. Observations of a galaxy from when the universe was just 1.2 billion years old have revealed a massive stellar bulge and a rotating disk. What's more, the galaxy appears stable morphologically despite undergoing a so-called starburst—where reams of stars form, which leads to a concomitantly larger-than-normal share of destructive supernovae—and possessing an active supermassive black hole that can likewise lead to scrambling. It's another case of early-universe galaxies confounding our expectations.
On the trail of solar-mass black holes
Kavli IPMU researchers have proposed a way to test further for the existence of solar-mass black holes, hypothetical objects with masses from approximately one to 2.5 times the mass of the Sun. Conventional models of the way stars evolve and die do not predict the formation of black holes with masses so small. Two other conceivable pathways for the creation of solar-mass black holes would involve neutron stars, the dense remnants of colossal stars that exploded as supernovae. The neutron stars could gobble up another type of hypothetical object, known as a primordial black hole and generated in the universe's earliest epoch, or still another hypothetical object, namely quantities of dark matter particles. In either case, this added mass could "transmute," as astrophysicists say, the neutron star into a heavier, denser solar-mass black hole. The Kavli IPMU researchers propose that if either of these pathways is viable, a population of solar-mass black holes should emerge observationally whose mass distributions closely follow that of regular ol' neutron stars. Astrophysicists already have tantalizing evidence of solar-mass black holes existing, courtesy of gravitational wave detections from LIGO that have identified a candidate 2.6-solar-mass black hole. Given the Kavli IPMU workup, though, that mass figure looks to be a bit too high for a solar-mass black hole to have arisen from the neutron star transmutation pathways.