Getting Down to the Brass Tacks of the Universe: Particles
by Adam Hadhazy
To understand why the universe is just so, Kavli researchers often deal with its most fundamental bits
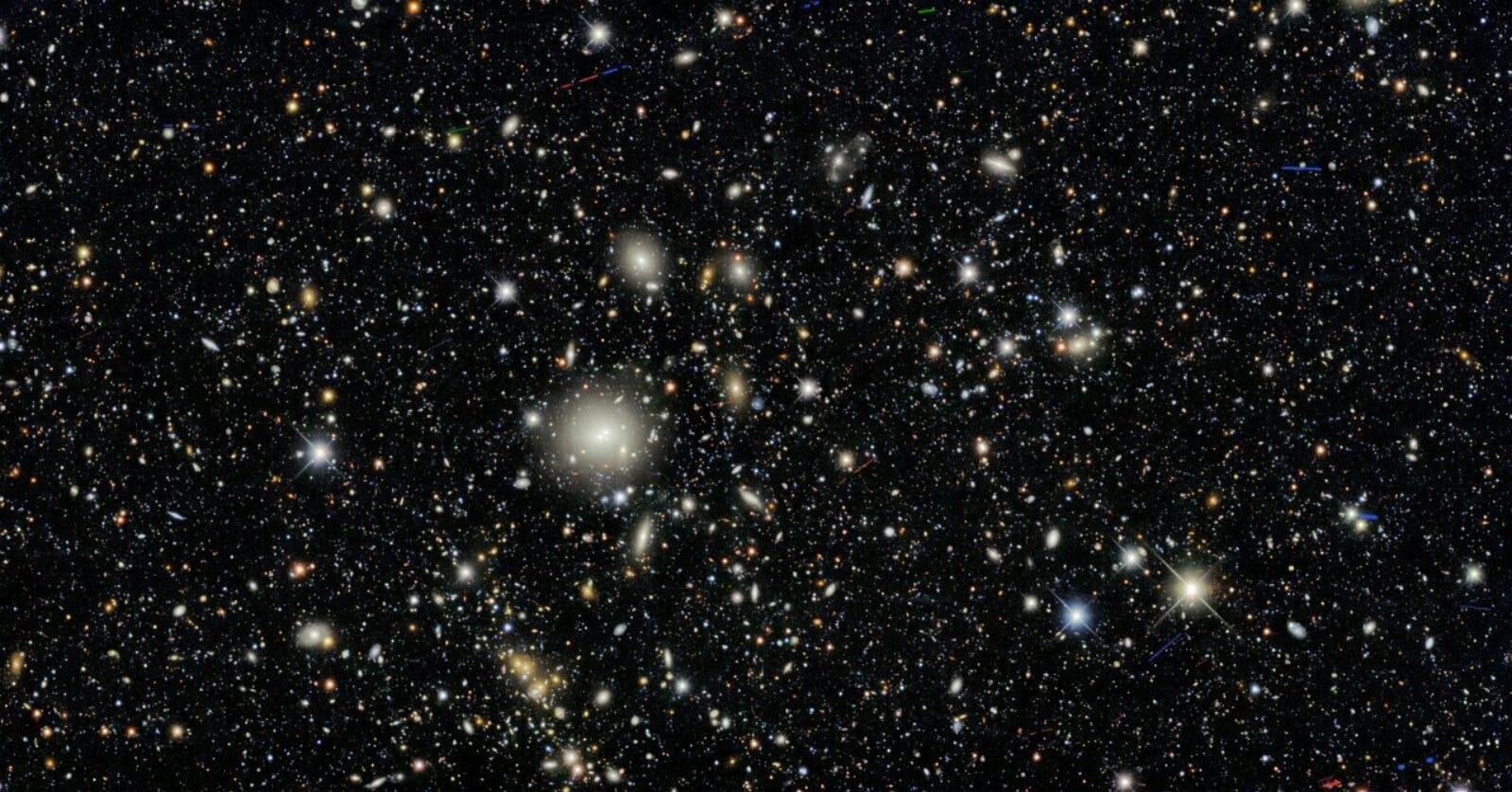
The Author
In the end (and also in the beginning), it all boils down to particles. Every material around us, as varied as the metal, glass, and plastic composing the very device upon which you read these words, is all made of the same stuff. We're not talking about the distinct atoms of each unique chemical element that comprises your device, such as aluminum, oxygen, silicon, hydrogen, and carbon, to name a few. No, boiling down still further, all those atoms are made of the same three particles—protons, neutrons, and electrons. (Excepting ordinary hydrogen, which has nary a neutron.) But there's yet another layer down. Those protons and neutrons are in turn fundamentally constructed of quarks—the brass tacks of everyday matter, from here to the edge of the observable universe. Many Kavli Institute-affiliated scientists happily concern themselves with the doings of reality at these elementary scales. It's part-and-parcel of better grasping how the universe at its grandest scales ultimately behaves as it does, stemming from how a single particle interacts with another particle. This work goes beyond the more "everyday" particles already discussed to less-heralded particle types, such as the muon, to theoretical particles such as the axion. The universe is indeed a playground of particles. Take a moment then, reader, as a constantly replenishing, slowly changing, and dolefully fleeting arrangement of roughly several octillion quarks to consider the particle nature of it all.
Biggest-ever map of galaxies released
A huge new batch of results from the Dark Energy Survey, a project that involves multiple researchers at Kavli astrophysics institutes, was announced recently. In a series of 29 papers, the DES researchers unveiled and analyzed the largest-ever maps compiled of galaxy distribution and shapes. The map covers more than 7 billion light-years. Overall, the map—based on three years of data from the five-year survey—strongly agrees with the so-called standard model of cosmology. This model is essentially a recipe for how matter, dark matter, and dark energy comprise the observable universe and explain its macroscopic properties. Early results from DES, though, along with other data suggest some discrepancy with the standard model, pointing to how far more observations and measurements will need to be taken to further cement our cosmological understanding.
Hypothetical particles could form a different kind of background radiation
Hitoshi Murayama from the Kavli Institute for the Physics and Mathematics of the Universe (Kavli IPMU) is one of three theorists suggesting in a new paper that hypothetical particles called axions might detectably exist alongside the universe's oldest light. That light, the cosmic microwave background, was generated when the universe became transparent to light some 380,000 years after the Big Bang. Murayama and colleagues have proposed that a Cosmic axion Background or CaB, could someday be discernable as well. Beyond confirming the existence of the axion, which is a highly attractive candidate for dark matter, detecting the CaB would provide astrophysicists with another kind of "fossil" with which to study the early universe.
Fast radio bursts by the dozens of dozens
Astrophysicists are souped that the Canadian Hydrogen Intensity Mapping Experiment, or CHIME, has recently quadrupled the number of known "fast radio bursts." FRBs for short, these blasts of radio waves pack in an astonishing amount of energy into just a few milliseconds' duration. Since the discovery of the first FRB in 2007, researchers have found less than 150 more—until CHIME, which in its year of observations from 2018 to 2019, piled on 535 more. Scientists at the Massachusetts Institute of Technology's Kavli Institute for Astrophysics and Space Research (MKI) have reported that the boatload of new FRBs come in two varieties: one-offs and repeaters. With the number of FRBs now large enough to start characterizing the overall phenomenon, researchers think that the two different kinds of FRBs likely have different generation mechanisms. Heavy, compact objects—namely neutron stars and black holes—are widely figured to be the sources of all FRBs.
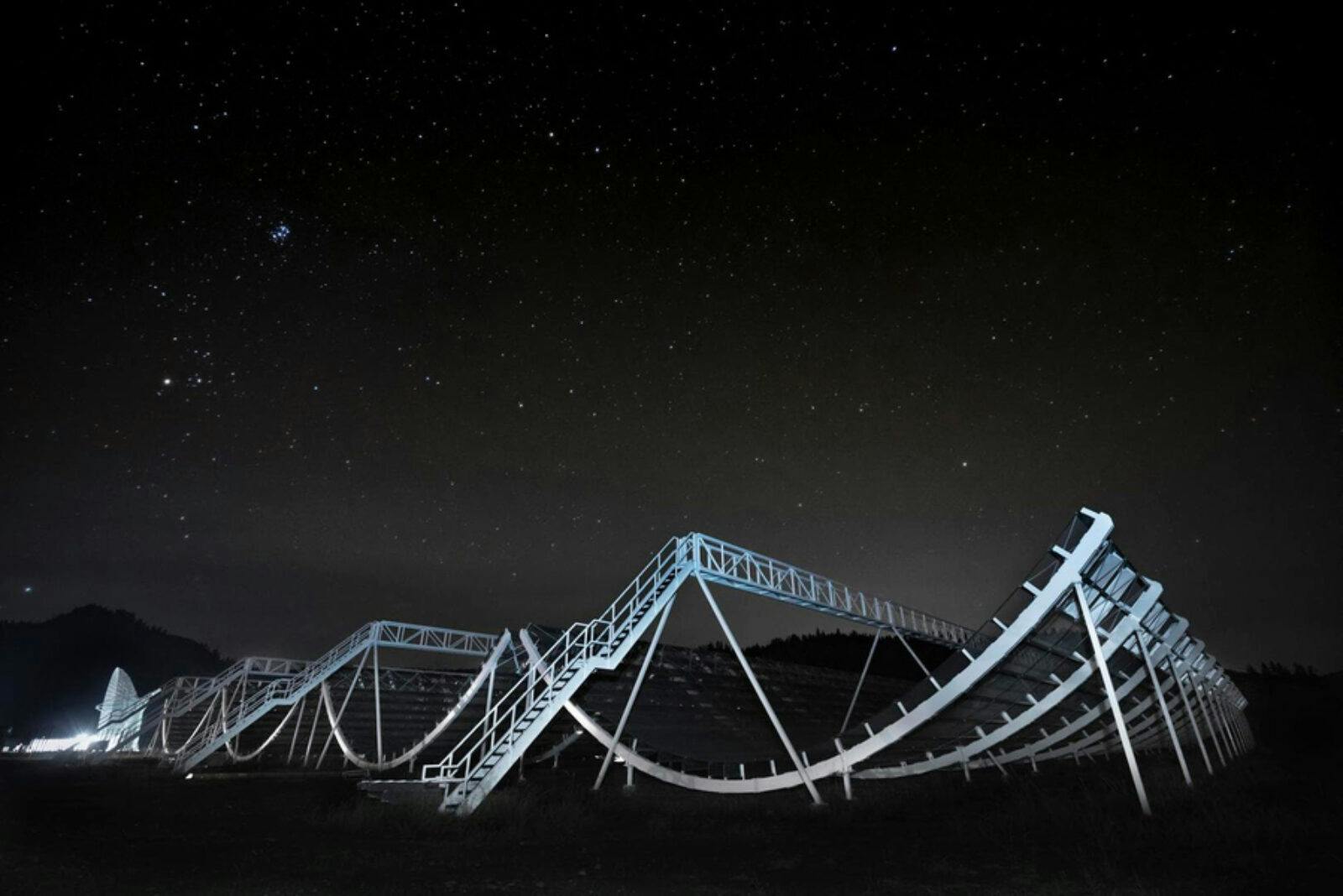
Muon's unexpected funkiness could help resolve fracas over universe' expansion rate
A little-known particle dubbed the muon made headlines in April when the Muon g-2 experiment reported that it surprisingly does not conform to the Standard Model of particle physics. Building off that discovery, Dan Hooper and Gordan Krnjaic—theoretical physicists at the Kavli Institute for Cosmological Physics (KICP) at the University of Chicago—have proposed that a tiny hypothetical particle, designated Z prime, could explain the muon's unexpected divergence, among other possibilities. Ultimately nailing down why the muon acts as it does could have implications for measuring the expansion of the universe, a presently hotly contested topic in cosmological circles. Calculating the expansion rate in part depends on accounting for and getting all the particular (that is, relating to particles) ingredients of the universe just right.
Weighing a neutron star, thanks to titanium
By measuring a variety of titanium in the leftover material from a stellar explosion, Kavli IPMU researchers and colleagues in a new study have inferred the mass of the white dwarf star responsible for the explosion. White dwarf stars, which are the aged remnants of stars like our sun, can accumulate extra matter from nearby stellar companions. That extra matter can eventually set off a runaway thermonuclear reaction that leads to a so-called Type Ia supernova. Scientists rely on shared properties amongst Type Ia supernovae to measure distances in the universe; the fainter the Type Ia, the farther away it must be, and in a measurable manner. The explosions are thus called "standard candles" in cosmology. However, the mass of the white dwarf in question in the new study was three times higher than the white dwarfs that typically blow up as these supernovae. The finding suggests there is a wider range of progenitor star property possibilities for Type Ia supernovae, in turn calling into question some assumptions about the "standardness" of standard candles.