'Heavy fermion' Pairing May Help Explain Superconductors
(Originally published by Cornell University)
July 15, 2013
CeCoIn5 is an oxymoron. It’s a “heavy fermion” material, in which electrons act like their mass is 1,000 times what it should be, because moving electrons interact magnetically with its atoms and slow down. But it also can be a superconductor, in which an electric current flows without resistance, when its super heavy electrons join in “Cooper pairs” that are magnetically neutral and avoid magnetic interference.
Since this and other heavy fermion materials were first observed some 30 years ago, scientists have been trying to figure out how this works; the answer could lead to high-temperature superconductors and a revolution in electrical engineering. Now Cornell researchers have, figuratively and literally, turned a microscope on the stuff and pried out its innermost secrets. Their results were reported June 14 in the advance online edition of the journal Nature Physics.
Next it’s up to the theorists, said J.C. Séamus Davis, member of the Kavli Institute at Cornell, the James Gilbert White Distinguished Professor in the Physical Sciences at Cornell and director of the Center for Emergent Superconductivity at Brookhaven National Laboratory. “We pretty much have enough information by comparing copper, iron, and now heavy fermion materials, to discern the fundamentals of high-temperature superconductivity,” Davis said.
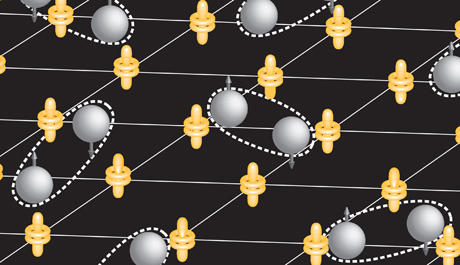
Recently discovered compounds of copper or iron with various trace elements will superconduct at temperatures as “high” as 150 Kelvins (degrees above absolute zero). Scientists have been frustrated in studying them because their electronic structure is extremely complicated, with electrons spontaneously arranging themselves in “stripes” and other patterns at the atomic scale. Heavy fermion materials are much simpler and offer a “third leg of the stool” to develop a general theory, Davis said, but the energies involved have been too small to measure until now. Davis acted in response to a suggestion from a group of German theorists who had heard of his work with an incredibly sensitive scanning tunneling microscope (STM) and believed it could measure the delicate energies involved.
The STM moves a tiny probe across a surface in steps smaller that the width of an atom. Freek Massee and Milan P. Allan, post-doctoral researchers in Davis’ group, used it to scan a sample of CeCoIn5 , a compound of cerium, cobalt and indium, cooled below 2.5 Kelvins, where it becomes a superconductor. In that state, Davis explained, the material is filled with a “sea” of Cooper pairs. By applying a positive charge to the STM probe and varying the voltage the researchers could determine how much energy is needed to pull an electron loose from a Cooper pair, a measure of the “glue” that holds the pair together. The “energy gap” varies for electrons moving in different directions: “If you travel northwest it’s easy to break the Cooper pair,” Davis explained, “but north or west it’s much harder.”
The research has produced a detailed map of the electronic structure of the material in its superconducting state – the first for any heavy fermion superconductor. Combining that information with the physical arrangement of magnetic atoms in the crystal, theorists may be able to figure out how the electrons become glued together, and then it might be possible to build a new compound with the same glue, but stronger, so the pairing could withstand higher temperatures, perhaps even up to room temperature.
Like most heavy fermion materials, CeCoIn5 must be cooled close to absolute zero with expensive and scarce liquid helium to become a superconductor, so it has no industrial value, Davis noted. “In terms of practicality it’s irrelevant,” he said. “ In terms of being [a model system] it's extremely important.”
The research was supported by the U.S. Department of Energy and the U.K. Engineering and Physical Sciences Research Council.