How Atomic Scale Devices Are Transforming Electronics
by Alan S. Brown
Manipulating electronic devices at the nanoscale is changing computing, but do we have the research infrastructure needed to commercialize today's latest research.
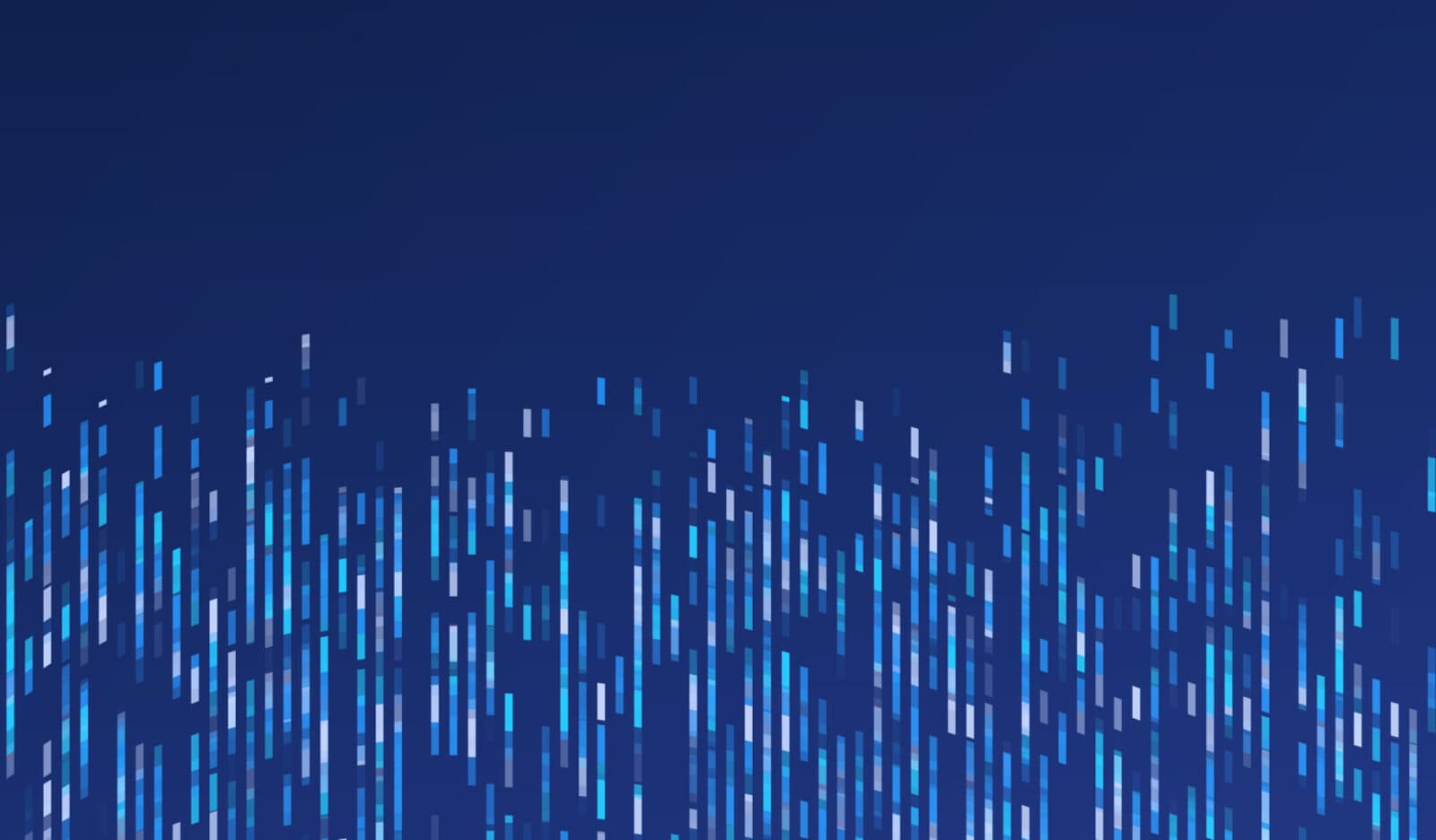
The Author
The Researchers
NANOSCALE ELECTRONICS BURST INTO THE NEWS at the start of 2012. Professor Michelle Simmons' lab announced it had created 4-atom-wide nanowires. IBM demonstrated it could store a bit of information on only 12 atoms, compared with 1 million atoms for today's most advanced hard drives. Then Prof. Simmons showed a working transistor made from a single atom. Meanwhile, Hewlett-Packard is planning to commercialize a mass-market flash memory device based on memristors, a new type of electronic device that stores information by manipulating the location of a few atoms.
Most news stories focused on the miniscule size of these new devices, and how they might keep Moore's Law (which states that shrinking chip device size doubles computing power every 18 months) hurtling through the next decade or two.
Yet there is another story here: These breakthroughs show how, after more than a decade of research advances, scientists and technologists are learning to measure and manipulate matter to create fundamentally different electronic devices.
The Kavli Foundation brought together three experts to discuss what makes those devices unique, how nanotechnology is likely to affect computing, and whether we have the research infrastructure necessary to commercialize today's latest nanoelectronic findings.
Participants
- Michelle Simmons, Scientia Professor and Director of the Australian Centre of Excellence for Quantum Computation and Communication Technology, University of New South Wales. She recently demonstrated single-atom transistors and 4-atom-wide nanowires.
- Paul Weiss, Kavli Professor at UCLA and Director of the California NanoSystems Institute. The Institute's goals are to advance and speed the commercialization of nanotechnology.
- Stan Williams, Hewlett-Packard Senior Fellow and director of the company's Cognitive Systems Laboratory. He is currently supporting efforts to manufacture mass-market memristor-based memory, build extremely large and sensitive sensor networks, and develop nanoscale devices that manipulate light.
The following roundtable discussion has been edited by the participants.
THE KAVLI FOUNDATION: Professor Simmons, your laboratory gained international attention earlier this year when you announced 4-atom-wide nanowires. Most researchers thought such small structures would show too much electrical resistance for practical use, yet that was not the case. Was this a surprise to you? And what do these wires imply about the future of atomic scale features?

The key to making these wires was combining scanning tunneling microscopy, a technique to image and manipulate individual atoms, with molecular beam epitaxy, a way of growing perfect crystals. It gave us great precision in all three dimensions, and when combined with a high density of the dopant atoms, allowed us to create these highly conductive nanowires.
PAUL WEISS: Because we increasingly have tools to create and to examine precise structures, I think we're going to see more and more demonstrations of what happens at the atomic scale.
We're developing our ability to make nanostructures the same way every time, then manipulate these structures so we can look at very small variations. This allows us to understand their behavior much better, and then come up with clever ways to recreate them. We're never going to make a billion transistors by manipulating individual atoms, but maybe we can use our knowledge to develop chemistries that recreate those structures.
STAN WILLIAMS: From a commercialization standpoint, the most important thing about nanowires and single-dopant transistors is that they demonstrate scaling. Nobody is going to invest hundreds of millions of dollars in a new foundry if they're not sure it's going to work. Michelle's work shows that there's a “there” there. It is a very important demonstration.
TKF: Stan, Hewlett-Packard and Hynix Semiconductor plan to commercialize a fundamentally new type of electronic device called the memristor, short for memory resistor. It works by manipulating only a handful of atoms to produce a dramatic change in electrical resistance. Information is stored by controlling those changes in resistance. The theory has been around since 1971. What made it possible to commercialize the technology now?

One important aspect of memristors is that they are simple structures made from materials and processes already used in semiconductor foundries. This is critical, because we would face tremendous resistance if we asked the industry to change technologies. But they don't have to change. If you know what you're doing - and there's a lot of intellectual property involved - literally any foundry could make memristors tomorrow.
In terms of commercialization, we'll have something technologically viable by the end of next year. It's sad to say, but the science and technology are the easy part. The economics, investment, and market readiness are harder. Our partner, Hynix, is a major producer of flash memory, and memristors will cannibalize its existing business by replacing some flash memory with a different technology. So the way we time the introduction of memristors turns out to be important. There's a lot more money being spent on understanding and modeling the market than on any of the research. Development costs at least 10 times as much as research, and commercialization costs 10 times as much as development. So in the end, research -- which we think is the most important part -- is only 1 percent of the effort.
TKF: I want to broaden that last question. What is driving progress in nanodevices today? Is it better tools? Improved measurements? Better models? Why are we making such progress now? What is the magic?
MICHELLE SIMMONS: From my perspective, there are two things. First, it's researchers having cross-disciplinary backgrounds. They do not just understand device measurements and device architectures, but also the chemistry and control of materials and the engineering to realize those devices. We're seeing more people who can see a device from beginning to end. This certainly had had a significant impact on our group in Australia.
The second is that we had an ambitious goal that pushed us. In 2000, we looked at Moore's Law, and realized it was just a matter of time before silicon devices reached the atomic scale. Just before this, we had Bruce Kane, currently a University of Maryland Fellow, working in Australia. In 1998, he published a seminal paper on how to build quantum computers in silicon using single atoms. It all converged, having the right people working together on the architectures with others with the technical skills to make single-atom devices. This led to discussions about the fundamental limits of silicon technology, whether we could build computers from single atoms, and how we would actually do that. Our government gave us long-term funding to start a center to pursue that goal.
PAUL WEISS: One of great things about the field is to ask what you want to know and to develop the means to find out. A lot of that comes from new tools and capabilities. When we first started moving atoms around -- we rewrote the software for a scanning tunneling microscope overnight at IBM to do it -- it was to find out what was underneath the atoms on the surface.

Our group likes to ask, 'What can't we do at the nanoscale? Is there some fundamental rule or law setting the limit?' Those conversations usually degrade into discussions about instrument minutia because, really, there is nothing stopping us but our own creativity and support for our research.
As Michelle pointed out, discovery takes time. In my own experience, it took 20 years to find a way to measure the tilt of a molecule. You chew and chew on those questions, but once you crack the door open, you get new insights and can ask further questions.
STAN WILLIAMS: To reiterate what Paul and Michelle said, there was no magic involved. People have worked their butts off for 10 years. Fortunately, they were supported all along the way.
In a lot of areas right now, though, the research support is very incremental and short-term. It's practically impossible to do something like Michelle talked about if you have a three-year timeframe, because at the end of that period, priorities might change and you might be forced to go off and do something completely different to get funding.
PAUL WEISS: I couldn't agree with Stan more about that. Sometimes, if you have money from an endowed chair, you can use it to keep long-term projects going. But it is a matter of stringing funds from different sources together, often in really awkward ways. You really have to commit and think this is the most important thing you can be doing.

STAN WILLIAMS: The memristor is not an overnight thing. We started working in this area in 1997. HP supported us, even when we went down lots of blind alleys, before we stumbled on the answers. It was a long-term strategic program, even when it wasn't at all clear we would be successful.
This commitment started when HP was still a chipmaker. We are not any longer. Our motivation is not to make memristors, but to ensure there is a supply chain. We're not going to make money off these chips. We are going to make money by building cool systems utilizing these chips. Hopefully, we'll build cooler systems than other people because we've been thinking about this technology longer.
TKF: We've mentioned memristors, nanowires, and single-atom transistors. What other new concepts jump out on your radar?
PAUL WEISS: I like two-dimensional materials, like graphene. You can produce them in atomically thin layers that give you transparency coupled with outstanding electronic and optical performance. You could use them for very fast photon detectors and extremely fast electronics.
STAN WILLIAMS: One area that's really exploding is nanophotonics.
MICHELLE SIMMONS: I was just going to say that.
We have such a long way to go. People talk about reaching the end of Moore's Law, but really, it's irrelevant. Transistors are not a rate-limiting factor in today's computers. We could improve transistors by factor of one thousand and it would have no impact on the modern computer." – Stan Williams
STAN WILLIAMS: The idea is to use nanoscale structures to control and direct light. We're working on that here, and there will be commercial products coming from this in the not-too-distant future.
TKF: What makes using light on chips so desirable?
PAUL WEISS: Light is a much faster way to communicate along a device than electrons. But the wavelength of light is much larger than the semiconductor structures we make now. People are used to moving light around with large-scale mirrors or splitters, things we lay out on an optical table. Investigators have come up with very clever ways to do that with nanoscale structures. If we can cut down the size of these structures by one, two, or more orders of magnitude, things start getting exciting about the speed and amount of information we can move on a chip or between chips.
MICHELLE SIMMONS: One application of this involves light-matter interaction. We are looking at encoding quantum information in photons of light with the aim of eventually sending it by optical fiber across a continent or the globe. Optical losses down the fiber limit the distance we can transmit information. So we need a way to replenish the signal. We are looking at the interaction between light and matter to develop components like quantum repeaters, which take light in a photonic state, store it in a solid state, and then pass it on as photons so you can extend the secure communications distance. Research in this area is really starting to happen now.
STAN WILLIAMS: Yes. At the data center scale, any time you want to move data more than a few millimeters, you do that as a photon. Our model is that communication is done by photons, computation by electrons, and storage by ions. Each has its limits. Ultimately, they will all be integrated together on a chip.
PAUL WEISS: The hard part until now has been photons. Now we are shrinking it to the same scale as everything else we are doing.
TKF: Will these nanodevices change how computers operate? Are we creating computers that are not just faster, but computers that behave in fundamentally different ways?

The rate-limiting parts are how you store and move information. These are visible targets and we know what we have to do to get there. We can continue to improve data centers and computers at Moore's Law rates -- doubling performance every 18 months -- for at least another 20 years without getting into something like quantum or neuronal computing.
That said, it's a lot of fun to think about building things that compute more like a brain, and we're doing some of that. But it will be 20 years before any of that is actually needed, because there are so many other improvements we can make first.
PAUL WEISS: If I had to guess, we're going to get better and better at adding new communications capabilities to our computers and phones, and at storing, moving and displaying information. We've gotten used to what our interfaces look like, so I think we're going to keep them but juice up the internals.
MICHELLE SIMMONS: For me, it is difficult to say because we're working towards developing a quantum computer. If we realize that dream, we will create something that works in a fundamentally different way, and hopefully the things that you can do with it will be fundamentally different.
There has been a lot of progress. People have built a few small demonstrator systems and have run algorithms on them. It looks very promising, but the fundamental question is whether we can maintain a coherent quantum state large enough to build quantum computers that will do things that classical computers just can't do in a timely fashion.
TKF: So far, we've been talking about nanodevices, like wires, transistors, and memristors. How do we put these devices together into systems? Where do we start?
STAN WILLIAMS: By the time you get into systems, people don't publish. I see that myself. Our group publishes about our devices, but practically nothing at the systems level. There's a good reason for that. Systems are where lots of know-how and differentiation come from. When things start to move into systems, it's like a black hole, they just disappear from sight.
The other thing I learned is that even the most revolutionary new technology has to be introduced as evolutionary because the market doesn't like disruption.
"[W]e're discovering new materials and new phenomena, we should be looking at how to integrate them into broader structures, to build robust systems... We can't ignore any one area. The beautiful part is when they start to come together." – Paul Weiss
MICHELLE SIMMONS: What Stan just said makes perfect sense. In the field of quantum computing, there is a company called D-Wave that sold the first commercial quantum computer to Lockheed Martin last year. It's very expensive and not everyone knows how it works. It will probably be a long time before you start seeing them sold in large numbers, but D-Wave is an example of a company that is trying to evolve them in the marketplace now.
TKF: Where should we be putting our research priorities going forward? Measurements? New devices? Integration of devices into systems?
PAUL WEISS: Yes, to all of the above. We've seen intriguing results in each of those areas. You'd be crazy to work on only one and ignore all the others. At the same time that we're discovering new materials and new phenomena, we should be looking at how to integrate them into broader structures, to build robust systems, and to manufacture everything reliably. We can't ignore any one area. The beautiful part is when they start to come together.
STAN WILLIAMS: I agree. Every attempt in the past to pick a winner and accelerate one thing has failed miserably. Although these recent breakthroughs look like miracles, they all took a decade or more to come to fruition. You have to make progress across a broad front. If we concentrate on one technology, we can move it forward. But once this one thing gets ahead of its support network, it's like pouring money down the drain because you don't have the tools or fundamental understanding or application knowledge. It doesn't work.
In the real world, scientific advances are built on strong, interconnected networks. If any one piece is gone, the other pieces don't work.

MICHELLE SIMMONS: I'm from Australia, where the overall pool of funding is small. Typically, what we do is choose the best people, let them work on something they are passionate about, and encourage them to be ambitious.
We have several ways to fund researchers. We have centers of excellence with ambitious goals that will have lots of spinoffs along the way. These receive longer-term funding. Our center, for example, received a seven-year grant last year, subject to a review at 4 years. We also have independent fellowships, where we pay the salary and help fund the research. This gives scientists a lot of time to do research and establish international collaborations.
We also receive funding from the United States Army. That funding has strict milestones we must meet every year. This combination of annual technology milestones and on-going funding for our center has enabled us to demonstrate significant advances every year.
PAUL WEISS: We don't have equivalent long-term funding, but there are funding mechanisms that help in a different way. When we put together a team to go after a new project, we often pick people who have worked effectively together on earlier projects. Then we bring in new blood by adding junior faculty and training students and post-docs.
It's a moveable party. We do not have the same long-term support as Michelle, so we improvise. We were able to sell the funding agencies on our nanotechnology center. One advantage in the United States is that there are multiple funding agencies, like NSF, DARPA and NIH. If one drops out of a promising project, for whatever reason, we can always seek funding from another, albeit with a shifted focus.
TKF: Nanotechnology has made many promises. Do we have the research infrastructure to deliver on them?
PAUL WEISS: We have a lot of people doing really good work. We have people that come here from all over the world. But now the funding sources, not just in nano but in life sciences and biomedical research, have not kept pace. The danger is that after all this investment, people might leave the field. If you have a terrific idea, you can still get it funded, though it might take a bit more work than in the past. It's the junior people who are having the most problems.
TKF: What about in the corporate world?
STAN WILLIAMS: The US National Nanotechnology Initiative [which funded nano research] has been huge in providing a reasonable number of people available in this area. I've hired at least 100 people over the past decade, and most of the younger people were able to study because their professors were supported by NNI. It played a critical role in creating today's multidisciplinary researchers.
That said, we simply don't have that diversity within the industrial research community any more. The fact is, with the exception of just a few companies, the industry in general is downsizing research. It's a period of retrenchment and consolidation, and there are fewer and fewer companies working in these areas now. Industry has less ability to try the ideas coming out of academic research.
We need competition. We need to try a lot of different things. There are not enough places performing research in industry to do that now. The entire enterprise seems to be winding down just at the point when the opportunities -- and their economic impact -- are growing more and more obvious.
"In the quantum computing world, we have developed the technology to make individual devices... In every field, there is a time to accelerate your commitment. In quantum computing, that time is now." – Michelle Simmons
MICHELLE SIMMONS: We're talking about making quantum devices for the future. As we realize new quantum technologies, we need to invest more money in people and equipment to turn them into useful products.
In the quantum computing world, we have developed the technology to make individual devices. We need to measure some of those devices for three months at a time to try to understand their quantum properties. We're limited by the amount of equipment we have to make the measurements, and we need more trained people who understand what those measurements mean. We could easily double our operation here if we had more money and people. In every field, there is a time to accelerate your commitment. In quantum computing, that time is now.
TKF: What do you think will be the most exciting news about nanoelectronics over the next year or two?
STAN WILLIAMS: We're starting to commercialize products based on the first decade of nanotech research. To stimulate further research investment, especially at the corporate level, we need a significant success or two to show that this funding can generate a huge return on investment.
MICHELLE SIMMONS: It's going to involve bringing together photons, atoms, and ions. I can't give you a specific example, but integrating that into a generic platform will provide the real excitement.
PAUL WEISS: I like surprises, and I hope there is a terrific one. For me, it would involve the most underdeveloped sector of the field, putting precise materials together at many scales to create functional materials.