Julia Greer: The Engineer as Materials Architect
by Alan S. Brown
Meet the director of the Caltech Kavli Nanoscience Institute
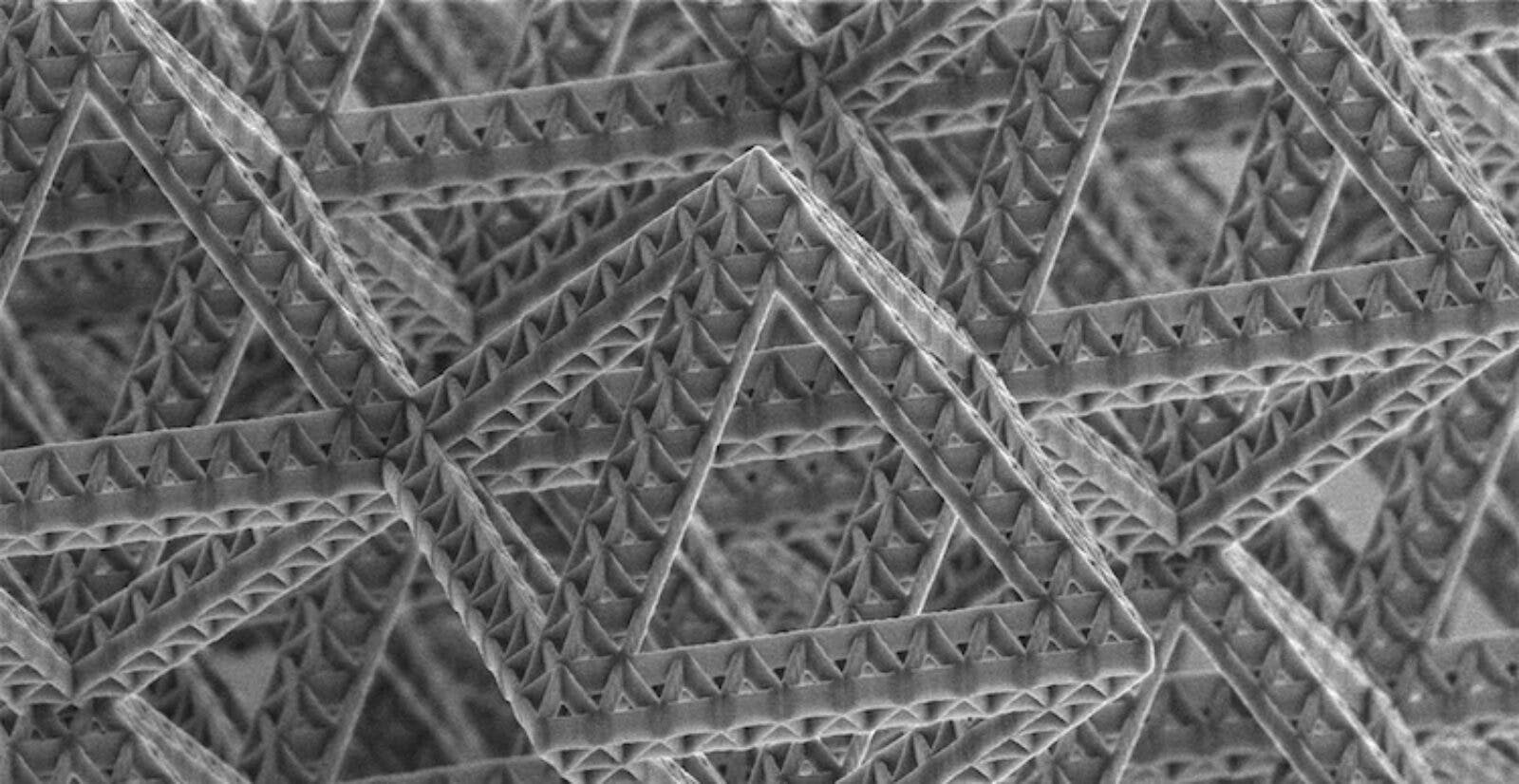
The Author
The Researcher
To appreciate the latest innovation from Julia Greer, the newly appointed director of California Institute of Technology's Kavli Nanoscience Institute, consider the natural sponges use for bathing or cleaning. In the store, they look like thin tan bricks only a few millimeters thick. Add water, however, and they suddenly swell to 10 or 20 times their previous size, revealing an intricate porous structure.
Greer, a professor of materials science, mechanics, and medical engineering, has done something similar, but with silicon. Silicon is ordinarily an extremely rigid and brittle material.
This time, her lab has created a nanoscale silicon lattice that, like a sponge, changes shape when given a jolt of electricity. The lattice's straight rods magically transform into S-shaped curves. Unlike the sponge, however, Greer can freeze the process at any point during the transition simply by turning off the electricity, and reverse it to recreate the original lattice.
It is an impressive feat of engineering, and also something more. It represents a new way of building materials that could one day lead to longer-lasting batteries and invisibility devices.
Hierarchies
Greer has always been a pioneer. Born in Russia, she emigrated to United States with her family at 16 and entered MIT— the only American university she knew — two years later. Once there, she drifted through chemical engineering classes while minoring in advanced musical performance.
At Stanford University for graduate school, she met Bill Nix, a professor who studied the mechanical properties of materials, and things changed.
"Only when I was given chance to do research and understand how satisfying it was to discover something that was not in a textbook did I develop a real passion for science," she said.
Working with Nix, Greer wrestled with understanding the mechanical properties of nanomaterials, which differed dramatically from larger versions of the same material. In the nano world, brittle materials might bend or interact with light, heat, or electricity in unexpected ways.
As she learned to synthesize nanoscale beams, rods, and other forms, Greer began building structures that would give her new ways to harness those strange properties. The result was what she and others call architected materials.
The bulk materials we see look solid, Greer explained. Put them under a microscope, however, and a different structure emerges. Once solid-looking ceramics have pores and metals have phases that look like tiny islands.
Architected materials add a new level of organization in between those two types of structures. Greer might, for example, start by synthesizing nanoscale rods or beams into a combination of trusses and arches like the ones found on the Eiffel Tower. Only Greer's Eiffel Towers started with building blocks many times smaller than the width of a human hair.
Even so, Greer could build them large enough to hold in her hand. They look, she said, like a "solid cloud" and weigh next to nothing.
Power
Over time, Greer learned to architect these materials from metals and ceramics. This made it possible to consider them for use in batteries.
The problem with today's lithium-ion batteries, she explained, is that they use carbon anodes that cannot store a lot of power. Silicon could store an order of magnitude more power — enough to power a cellphone for a week — but it is brittle. All anodes expand when they interact with lithium to store power. Silicon is worse than most. It triples in size, which causes it to crack and fail. Researchers tried making silicon in all sorts of different shapes, but they all broke. A graduate student suggested that working with a structure that was mostly air would give silicon more room to expand without cracking.
Ultimately, Greer and a team led by graduate student Xiaoxing Xia developed a material that expands and contracts when interacting by interacting with lithium. By building flaws into the architected structure, Greer's lab was able to control exactly how those changes progressed.
"Nanomaterials are very sensitive to defects," she said. "So we took a flaw and called it a feature."
The technique appears to work, though it may take years for it to work its way into batteries at affordable prices. Yet the work is important because it shows the power of architected materials to do things that would be impossible otherwise.
The new material has other potential uses as well. Its nanoscale elements are small enough to interact with light to make sensitive optical instruments. It could even bend light to render objects invisible. Just as water changes a natural sponge, architected materials open the door for new pathways in materials discovery