Meet the Toolmakers
by Lindsay Borthwick
At the Kavli Futures Symposium, the future of neurotechnology never looked so bright.
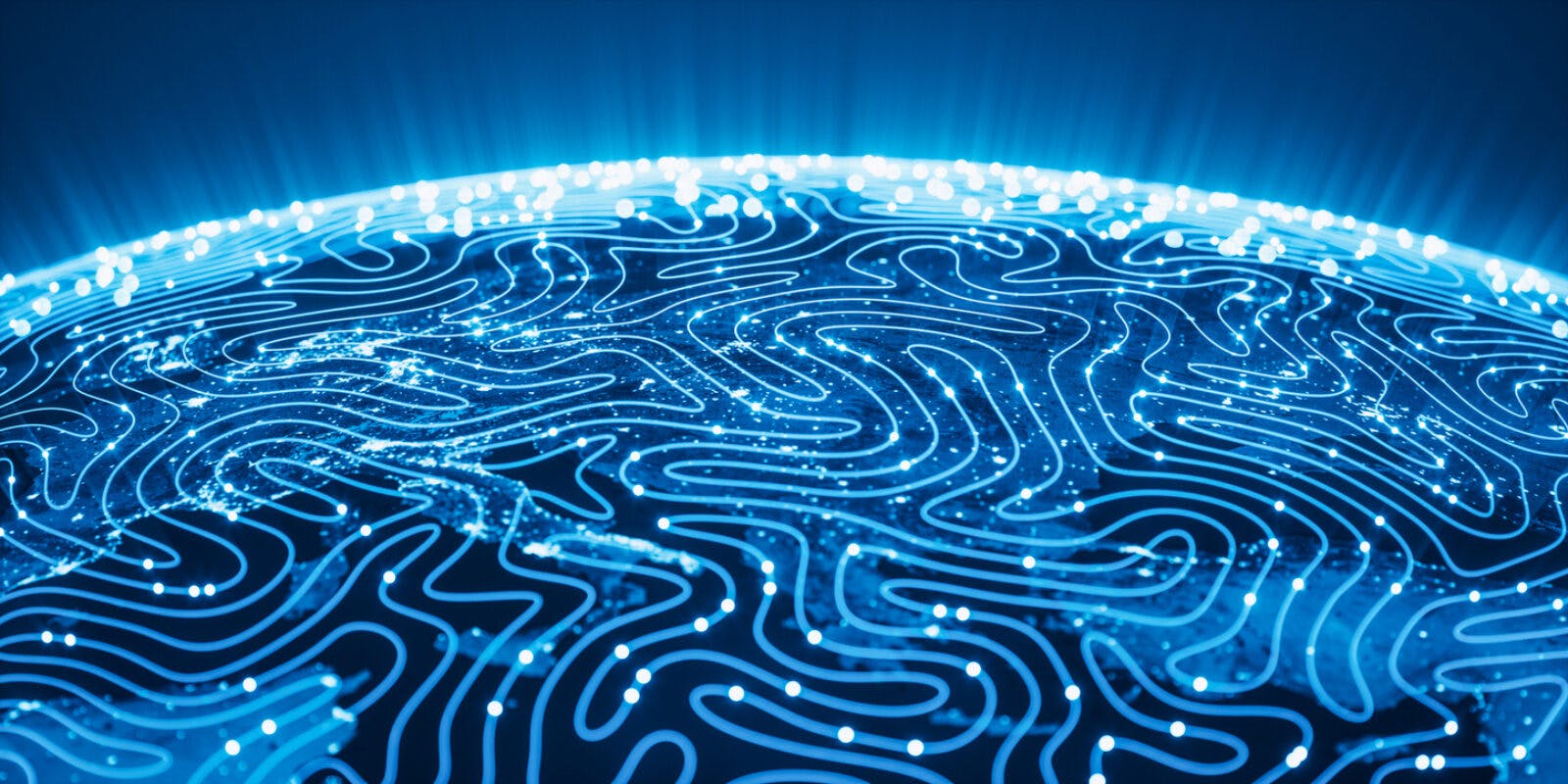
The Author
The Researchers

Cathy is paralyzed from the neck down due to a stroke. Until that day, she hadn’t taken a drink unaided for 15 years. But with the help of a pill-sized device implanted in her brain, she was able to instruct a robotic arm to grasp a bottle and move it to her mouth.
The achievement brought a smile to Cathy’s face. It also demonstrated the emerging power of neurotechnology, a rapidly developing field that merges neuroscience and engineering to create new tools to treat the brain and study how it works.
The experimental device that reads out and translates signals from Cathy’s brain is one of nine brain-computer interfaces, known as BrainGate, which have been implanted in individuals with paralysis to reconnect their brains to their bodies. It is the invention of a large team of collaborators led by John Donoghue, Director of the Brown Institute for Brain Science in Providence and a world leader in combining neuroscience and engineering.
Donoghue was one of a multidisciplinary group of 16 scientists who spoke at the Kavli Futures Symposium: The Novel Neurotechnologies that Rafael Yuste called “a dazzling feast of exciting ideas.” Yuste is Co-Director of the Kavli Institute for Brain Science at Columbia University in New York and the Director of the new NeuroTechnology Center, which hosted the two-day event late last year.
That’s not surprising given that that human brain is, as one presenter at the symposium put it, “insanely complex”—consisting of about 85 million neurons that form trillions of connections. It is also largely uncharted.
The Kavli Futures Symposium provided a sneak preview of the research tools that may soon help unravel this complexity.
Mapping the Brain
When President Obama mentioned the brain in his 2013 State of the Union Address, the word brain reverberated through the research community in the United States and abroad. What would a renewed Federal investment in brain research look like?
The answer became the Brain Research through Advancing Innovative Neurotechnologies (BRAIN) Initiative, a bold 12-year effort to map the circuits of the brain and understand how they function. Enter neurotechnology. As the “N” in “BRAIN Initiative” makes clear, mapping the brain’s complex circuitry will require tools to take even better measurements of the brain than neuroscientists can take today.
The challenge is largely one of scale. For more than 50 years, neuroscientists have been studying the activity of nerve cells, the basic building blocks of the brain, one at a time. For the past 20 years, in contrast, they’ve been able to zoom out and observe the activity of the whole brain, using imaging techniques such as functional magnetic resonance imaging (fMRI). What they need are tools that work somewhere in the middle, at the level of circuits. After all, nerve cells, or neurons, don’t exist in isolation.
“Between a single neuron and the millions of neurons represented in a single voxel of a fMRI [scan] is really where the action in the nervous system takes place. And that is the area where the field needs to make progress,” said Cornelia Bargmann, a Rockefeller University neuroscientist and Kavli Prize laureate, at the symposium. Bargmann also co-chaired the advisory panel that established the BRAIN Initiative’s scientific priorities.
The time is right, she added, because a variety of technological advances in genetics, optics, electronics and nanotechnology have already occurred that are narrowing that measurement gap.
But what does it really mean to map the brain? Neuroscientists are actually working to create two distinct but complimentary maps. One aims to capture the brain’s structure, namely its cells and their myriad connections, in exquisite detail. The second is a functional map detailing the electrical signals, or neural activity, that flow along these connections, carrying information from one part of the brain to another as we think, feel or move.
Here, we look at some of the mapmaking tools introduced at the Kavli Futures Symposium that may soon help chart the brain.
Anatomy of a Circuit
It has been more than 100 years since the celebrated neuroanatomist Santiago Ramón y Cajal produced his finely detailed drawings of different types of neurons. Yet neuroscientists still don’t have a complete parts list for the brain, let alone a wiring diagram of their connections.
What’s clear is that there are at least several hundred types of neurons in the mammalian brain that vary in terms of shape, size and function, and that new types are still being discovered.
Neuroscientists are also slowly generating the “connectome,” that much-needed wiring diagram, in humans, mice and other model species. They’re doing so by slicing the brain as thinly as possible, staining it and using electron microscopes to see its structure. The trouble is, these slices need to be aligned in order to reconstruct the neurons and their fine projections in three dimensions, which is an enormous computational challenge. Brain cells are also densely packed, which makes them hard to distinguish. Cajal called the brain's dense thicket of cells an “impenetrable jungle.”
So how are neuroscientists finding their way through it?

To date, more than 160,000 people from 145 different countries have played the game. But progress is slow even with a legion of talented volunteers. For example, this year his lab is attempting to create one of the most extensive catalogs of cells in the retina using EyeWire. If they succeed, they will reconstruct only about 350 retinal cells in a block of tissue that is 200 microns cubed (about twice the width of a human hair). In order to eventually map parts of the cortex, a much larger and more complex brain region, Seung will need computers that can automatically trace the connections quickly and accurately. At the moment, however, humans perform the task better than computers do, so he is using the data they generate to improve the machines’ performance.
As a theoretical neuroscientist, Seung isn’t just interested in creating detailed maps. He is also using the anatomical information the connectome contains to develop theories about how the brain works. For example, by analyzing the structure and connections of starburst amacrine cells, a type of neuron found in the retina, he has proposed a model for how these specialized cells detect motion—something that has taken 50 years for neuroscientists to figure out.
Like Seung, neuroscientist Konrad Kording from Northwestern University in Chicago is working with connectome data from the mouse retina. His focus is on trying to automate the discovery of cell types and their local circuits using mathematics. At the symposium, he described a statistical method that can identify different cell types about as well as human anatomists can, and much more accurately than do existing methods. His key advance is to factor in some of the rich structural data about neurons and their connections that is captured by connectomics, such as the distance between neurons. Distance is an important variable because it’s likely that the farther apart two neurons are, the less connected they are, and vice versa.
With the tide of new brain mapping tools comes a tidal wave of data, and neuroscientists need ways to manage those data and extract meaning from them. At the symposium, Kording argued that the field is in need of an informatics revolution akin to the one that followed the advent of genome sequencing techniques more than a decade ago. “We’re getting a lot of data but we ultimately need to make sense of it and get at models, at principles about the brain,” he said.
The Dynamic Brain
In addition to mapping structure, researchers are inventing new ways to monitor these circuits at work, coursing with electrical activity.
This goal was considered impractical just a few years ago. For decades, neuroscientists were limited to monitoring the electrical activity of single neurons using an electrode. Now, by packaging electrodes together, they can monitor the activity of a few hundred neurons at once. These neural probes can be surgically implanted into lab animals, like mice, that are freely exploring their environment, which allows researchers to study how the brain processes information as they sense their surroundings, make decisions and execute movements in real time. And, as John Donoghue’s work shows, these probes can also be married to the human brain to assist people with paralysis.
In fact, it’s remarkable that with a device like BrainGate, which intercepts the electrical signals from about 200 neurons, Cathy can move a robotic arm. Imagine what might be possible if researchers could read out the activity of tens of thousands or millions of her neurons at once.
Nanotechnology is on the verge of delivering just that, according to several researchers at the Kavli Futures Symposium.
Some, like Tim Harris, Director of the Applied Physics and Instrumentation Group at Janelia Research Campus in Ashburn, Virginia, are pushing neural probe technology to the limit by miniaturizing it. Working with a European nanoelectronics research organization called Imec, he is taking advantage of microfabrication techniques developed by the semiconductor industry to create silicon-based probes that can record electrical activity across a wide area of the brain. The project, still in its test phase, has produced arrays of nearly 500 electrodes, or channels, to record signals from a mouse brain. The probes are expected to be available to brain researchers in 2016.

If Roukes is correct that 100,000 cells is the upper limit for silicon-based probes, what are the alternatives?
Charles Lieber, a nanoscientist from Harvard, may have one. He has pioneered a way of integrating electrical sensors and their wires with living cells to create “cyborg” tissues. For example, when he cultures heart cells with electronic sensors in a dish, the two components become enmeshed, and the sensors can actually read out information from the heart cells, such as the rate at which they beat. The technique works with neurons, too. Lieber has embedded the sensors in a flexible polymer and injected them into the brain and retina to record the electrical activity of nerve cells. The polymer unfolds and integrates with the surrounding brain tissue without causing much damage, a vast improvement over rigid electrodes.
Twinkling Neurons
However, Roukes is betting on an altogether different technique—one that uses optics rather electronics to monitor brain activity. Called “integrated neurophotonics,” he is developing it with support from the BRAIN Initiative.
Unlike electrodes which track voltage changes in a cell, optical tools for mapping brain activity track fluctuations in calcium levels. When a neuron is active, fluorescent proteins, which have been engineered into the cells and are sensitive to calcium, glow more brightly. Neuroscientists can use various kinds of light microscopes to observe these cells as they light up, simultaneously recording the activity of thousands of neurons. In 2013, for example, a team of researchers monitored the activity of 80,000 neurons in a live zebrafish larva using a new optical technique. It was a remarkable achievement.
But researchers need to overcome several limitations of optical tools if they are to succeed at mapping the brain in action—a central goal of the BRAIN Initiative—in larger animals. The main issue is that light microscopes can only see into the superficial layers of the brain, making it literally impossible to monitor its inner workings. (This isn’t an issue with zebrafish larva, which are transparent.) Secondly, most microscopes aren’t fast enough to capture brain activity in real time.
Integrated neurophotonics overcomes the problem of depth by putting the hardware that is needed to detect the fluorescent glow of active neurons right into the brain using ultra-thin probes. These probes are still be packed together into arrays but far fewer of them are needed to monitor neural activity, making it feasible to monitor hundreds of thousands or even millions of neurons.
“Getting good images deep in tissue is the biggest impediment in optical imaging," said Jerome Mertz, a physicist from Boston University who also presented at the symposium. But “I think the field is on the verge of something very big that will allow us to go arbitrarily deep in tissue.”
Mertz was referring to recent fundamental breakthroughs in optical imaging that may eventually help brain researchers. But in the meantime he has some bright ideas of his own. What if neuroscientists could peer into parts of the brain that are difficult to access with light microscopes just by using an optical fiber? In fact, Mertz has shown that it’s possible to see light-emitting objects through a fiber that is thinner than a human hair.
Fibers usually scramble spatial information, obscuring an image. But Mertz has figured out a way to transmit images through a fiber, using a technique borrowed from the telecomm industry, by converting spatial information into color and then back again. Though the technique is just at the proof-of-concept phase, it represents the kind of out-of-the-box thinking that neuroscience needs to realize its ambitious goals.
Riding the Wave
The presenters at the Kavli Futures Symposium underscored that the field of neurotechnology is rapidly coming of age, spurred by ingenuity and by investments such as the BRAIN Initiative. Daryl Kipke, an engineer and CEO of neural-probe manufacturer NeuroNexus, put it simply: “It’s finally cool to be a toolmaker.”
Thanks to new brain-mapping tools, neuroscientists can measure the brain in unprecedented detail. With other new neurotechnologies, they can also, for the first time, manipulate the brain's circuits. This not only allows them to test theories about how the brain functions but may also lead to new interventions similar in promise to BrainGate.
“The neurotechnology future is exceptionally bright,” concluded BrainGate’s John Donoghue. “The devices we can have both sensing and stimulating [the brain], and also integrating them together, will provide us with many new ways to treat human disorders and, importantly, to investigate the human nervous system in ways that we have never been able to do before.”