One Promising Puzzle Piece for Confirming Dark Matter Now Seems Unlikely Fit, Researchers Find
New research adds doubt that a prominent European astrophysics experiment may have found evidence of dark matter. In an interview, Stanford’s Stefan Funk and Justin Vandenbroucke talk about their findings, as well as how they tricked a telescope into searching for particles it wasn’t designed to detect.
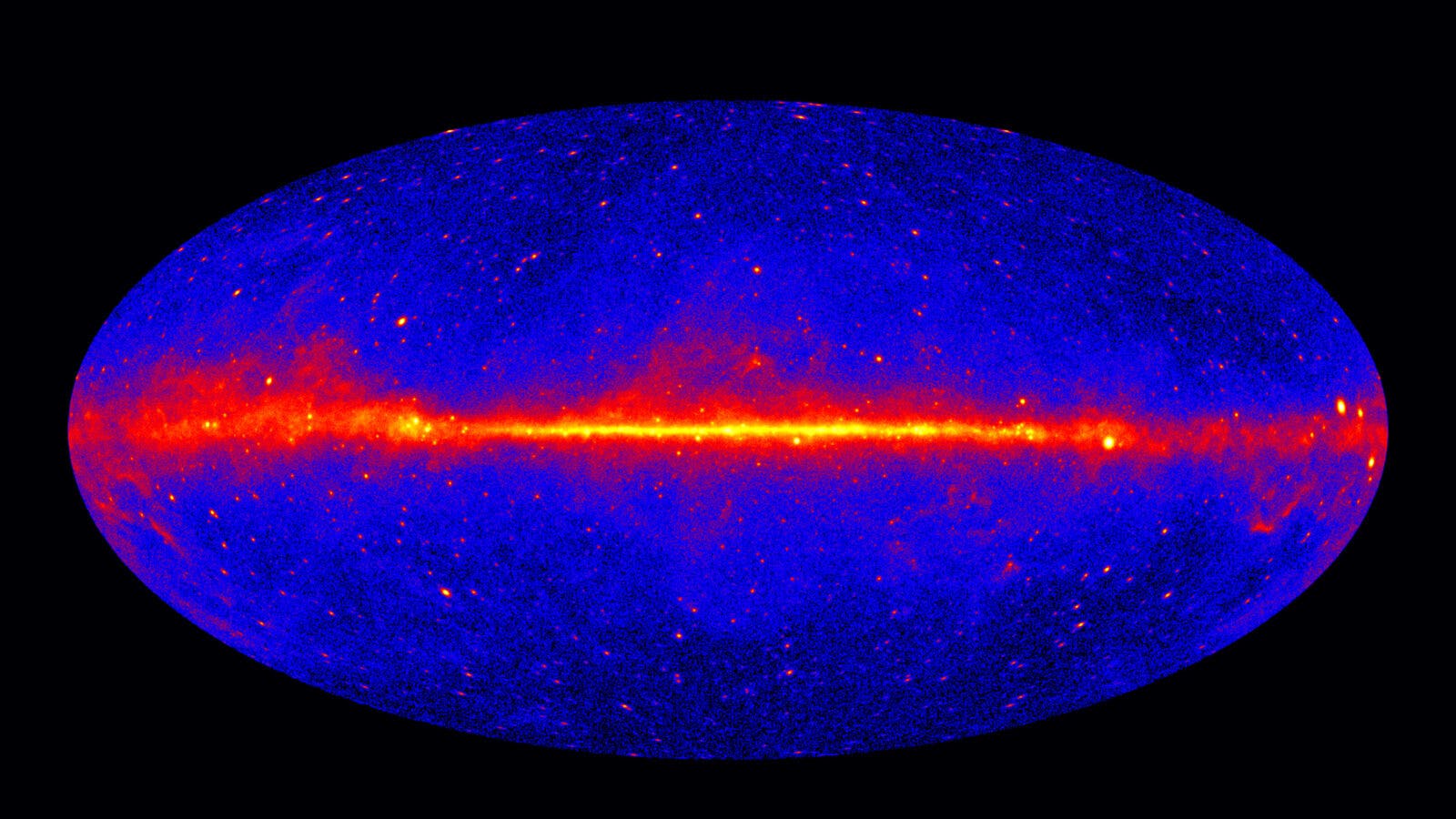
The Researchers
LIKE JAZZ MUSICIANS who make up a melody as they go along, scientists often improvise even after an experiment is underway. One recent example of this comes from the Fermi Gamma-ray Space Telescope. Launched by NASA in June 2008, the $690 million telescope has since been working as advertised, providing scientists with the most complete look yet at gamma rays, the highest energy forms of light. But just two months after the launch, a tantalizing finding from a European experiment hinting at evidence of dark matter had Stefan Funk and Justin Vandenbroucke wondering if the telescope could be used to look at something for which it wasn’t intended -- specifically, electrons and their antimatter twins, positrons, that are streaming across the universe in cosmic rays.
Their problem was that the telescope, designed to detect neutral gamma rays, doesn’t have a magnet for separating negatively charged electrons and positively charged positrons. So Funk, Vandenbroucke, and Stanford graduate student Warit Mitthumsiri, all at the Kavli Institute for Particle Astrophysics and Cosmology at Stanford University, started looking beyond the experimental hardware. They found a magnet a few hundred miles away from the telescope that might do the trick. It happened to be the Earth itself, which, thanks to its magnetic field, bends the paths of charged particles raining more or less continuously from space. (The spectacular aurora visible at high latitudes is the result of charged particles being bent and funneled toward the poles and impacting the Earth’s atmosphere.)
After studying up on geophysics maps and calculating precisely how the Earth was filtering out charged particles seen by the telescope, the researchers went ahead with their analysis, and wound up with somewhat dramatic results. Their paper, submitted to Physical Review Letters and originally published on the internet physics archive on Sept. 2, confirmed the curious excess of antimatter positrons formally reported in the 2009 study in the journal Nature that had the physics world agog. However, Funk and Vandenbroucke’s analysis is most noteworthy for what it didn’t see -- a sudden drop-off of this excess in those cosmic rays beyond a certain energy level, as many theories predicted would happen if dark matter was involved. Their result casts doubt on the dark matter explanation, which is one reason why the paper started making news just four days after it was published online. The first scholarly paper on the implications of Funk and Vandenbroucke’s work appeared on the physics archive soon thereafter. That paper declared that “the standard positron production scenario must be incomplete.” In other words: Who knows where these excess positrons are coming from?
Funk, an assistant professor at Stanford and member of KIPAC, and Vandenbroucke, a postdoc and Kavli Fellow, recently talked to The Kavli Foundation about improvising in the big-budget world of experimental physics and throwing cold water on a hot theory about dark matter. Here are the highlights of that conversation.
An Interview with Stefan Funk and Justin Vandenbroucke
THE KAVLI FOUNDATION (TKF): Let’s start with the finding from Europe that inspired your work. Preliminary data were shared earlier, but in 2009 researchers working on the Payload for Antimatter Matter Exploration and Light-nuclei Astrophysics, PAMELA, reported more antimatter than expected in our galaxy, a result that had been shown in earlier experiments, as well. What might this excess antimatter have to do with dark matter?
STEFAN FUNK: There are various theories, but the basic idea is that if a dark matter particle were to meet its anti-particle, both would be annihilated. And that process of annihilation would generate new particles, including positrons. When the PAMELA experiment looked at the spectrum of positrons, which means sampling positrons across a range of energy levels, it found more than would be expected from already understood astrophysics processes. The reason PAMELA generated such excitement is that it’s at least possible the excess positrons are coming from annihilation of dark matter particles.
TKF: You confirmed this excess, and showed that ratio of positrons to electrons continues to increase at higher and higher energy levels. Energy level is basically synonymous with speed, so the faster the cosmic rays are travelling, the more positrons exist for every electron. Why is this interesting?
FUNK: There are two reasons. First, recall from Einstein’s famous e=mc2 equation that energy (e) and mass (m) are effectively the same thing. If the antimatter we measure is coming from the annihilation of dark matter particles, then the positron excess should drop off fairly suddenly at an energy level that corresponds with the mass of the dark matter particle. In fact, we found that the positron fraction increased continuously, right along with increasing energy level. Some have concluded that this altogether rules out dark matter as a source of the antimatter we’re measuring. At the very least this means that if the positrons are coming from dark matter annihilation, then dark matter particles must have a higher mass than allowed by the PAMELA measurement.
I would also point out that it’s already well understood that positrons are produced when protons in cosmic rays interact with other interstellar material. We’re talking everyday matter here, not dark matter. The faster these protons are traveling, thus limiting their interactions, the fewer positrons should be produced through such processes, at least according to accepted theory. But that wasn’t the case. We saw more positrons at these faster, higher energy levels, which is curious.
TKF: So, are you willing to say that the positrons detected by both your work and the PAMELA experiment aren’t coming from dark matter?
JUSTIN VANDENBROUCKE: We're taking an observational point of view and simply reporting the data that we observe. However, I know that articles are already appearing that say our result likely rules out the dark matter interpretation. Personally, I think that is too strong of an interpretation.
TKF: You did all this work using a telescope designed to measure something else entirely. Specifically, gamma rays. Are there other examples in physics like this -- of effectively sticking a square peg in a round hole and making it fit -- or are you guys trailblazers of sorts?
VANDENBROUCKE: There is a similar example from neutrino physics. In the 1980s there was an experiment at Japan’s Kamioka Observatory called Kamiokande. It was designed to see if protons, thought to be stable, might decay over very long periods of time. The experiment’s very large detector turned out to be a good neutrino detector, too, and researchers wound up using Kamiokande and its successor, Super Kamiokande, to detect neutrinos from the Sun, the atmosphere and even from a supernova. That work earned Masatoshi Koshiba the Nobel Prize in Physics in 2002.
TKF: Impressive. Still, aren’t you making your life more difficult than it needs to be? Wouldn’t it have been easier to do this work on a detector designed to separate charged particles?
FUNK: I find it to be fascinating to try to get the most out of an astrophysical instrument and I think we did that with this measurement. It was very satisfying that our approach, novel as it was, seemed to work so well. Also, you really have to go where the science takes you. Our motivation was to confirm the PAMELA results because they are so exciting and unexpected. And as far as understanding what the universe is actually trying to tell us here, I think it was important that PAMELA results were confirmed by a completely different instrument and technique.
VANDENBROUCKE: The thing that was most fun about this analysis for me is its interdisciplinary nature. We absolutely could not have made the measurement without this detailed map of the Earth's magnetic field, which was provided by an international team of geophysicists. So to make this measurement, we had to understand the Earth's magnetic field, which meant poring over work published for entirely different reasons by scientists in another discipline altogether. The big takeaway here is how valuable it is to measure and understand the world around us in as many ways as possible. Once you have this basic scientific knowledge, it’s often surprising how that knowledge can be useful.
TKF: Are there other examples of interplay between astrophysics and geophysics?
VANDENBROUCKE: Neutrino physicists are starting to measure “geoneutrinos” generated by radioactivity in the Earth's crust. These can help measure various aspects of radioactivity, including how much there is in the crust and how much it contributes to the Earth's heat. Another example is a neutrino detector at the South Pole, IceCube. The deep holes that have been drilled for it have also been used to produce detailed records of the climate going back tens of thousands of years.
TKF: Okay, so the bottom line is there is an excess of positrons, at least in our neighborhood of the universe, and researchers aren’t sure why. Dark matter might be, though probably isn’t, the explanation. Are there other experiments out there that will shed more light on this?
VANDENBROUCKE: There's an important one called the Alpha Magnetic Spectrometer, AMS. It was carried to the space station in May 2011 and it's been running since then. That detector should be able to make this measurement with more precise detail and at higher energies.
FUNK: AMS has a very large magnet in its detector and so can naturally and very easily distinguish between electrons and positrons. That experiment will most likely be able to make a final statement on this. It’s something we are all eagerly awaiting.