The Latest from LIGO
by Adam Hadhazy
As Earth's premier hunter of gravitational waves, LIGO is making a killing
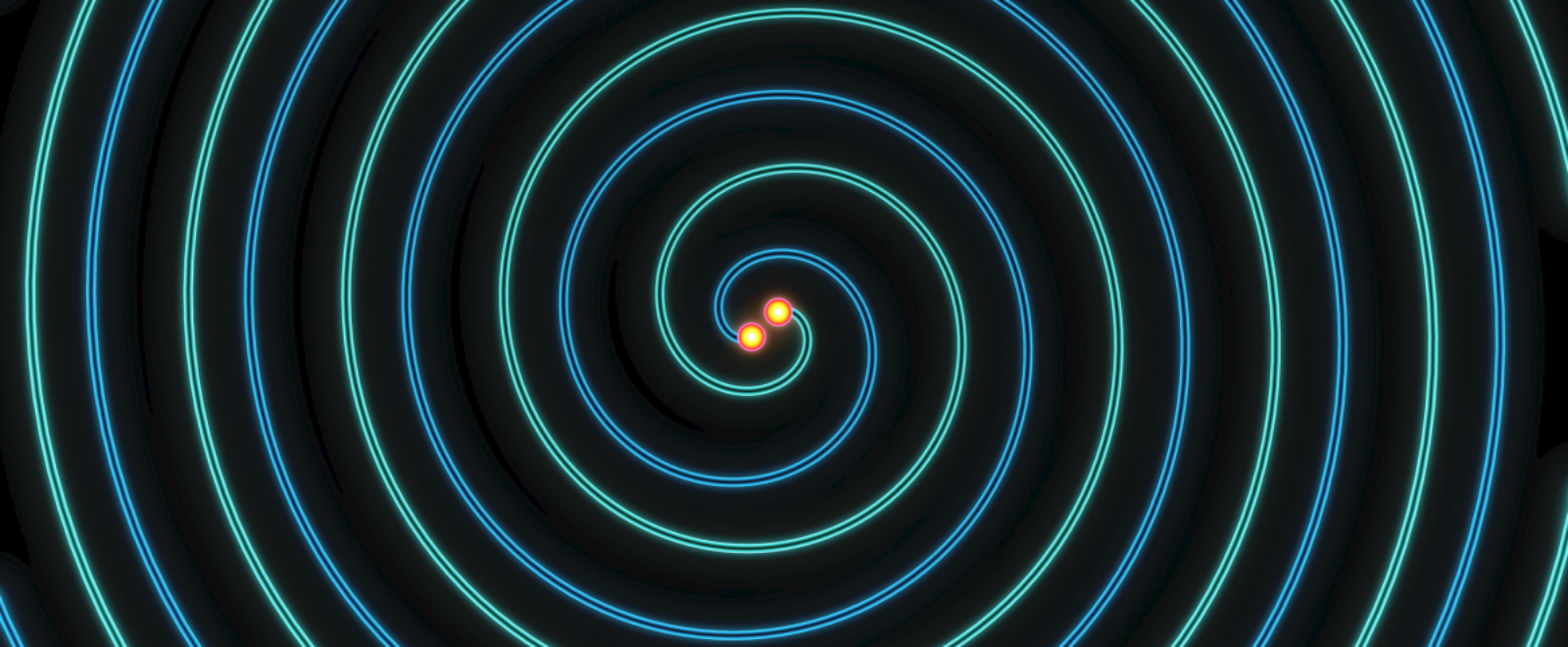
The Author
It's heady days for the fledging field of gravitational wave science. A century after Albert Einstein first predicted gravitational waves' existence, these elusive ripples in spacetime finally were directly detected in late summer 2015. The instrument that made the breakthrough, the Laser Interferometer Gravitational-wave Observatory (LIGO), along with its European partner, VIRGO, has since made nearly two dozen detections, opening up a revolutionary new way to study the cosmos.
LIGO is presently in its third observing run, having received a set of sensitivity upgrades and then rebooted on April 1, 2019. Those upgrades have paid off, with the twin LIGO detectors—located in remote regions of Louisiana and Washington State—now churning out a new detection on average once per week.
Even with gravitational wave capturings becoming routine, the team of hundreds of scientists behind LIGO have not grown calloused to the momentousness of the discoveries.
"It is definitely still exciting," says Peter Fritschel, the director of LIGO at the Massachusetts Institute of Technology (MIT) and a member of its Kavli Institute for Astrophysics and Space Research (MKI). (MIT and Caltech jointly built and operate LIGO.)
"I get text alerts whenever a significant trigger has come in," Fritschel goes on, "and I still go into our database as soon as I can to see what kind of event it is."
Gravitational waves form when massive objects accelerate, sending out ripples across spacetime like a rock dropped in a pond. The collisions of ultra-dense black holes, the famously invisible entities from which even light cannot escape, are the wellspring of most of the gravitational wave events recorded to date. In these cataclysmic collision events, a pair of black holes whirl initially around each other at tremendous speeds before merging, converting Suns'-worth of mass into gravitational energy in the process.
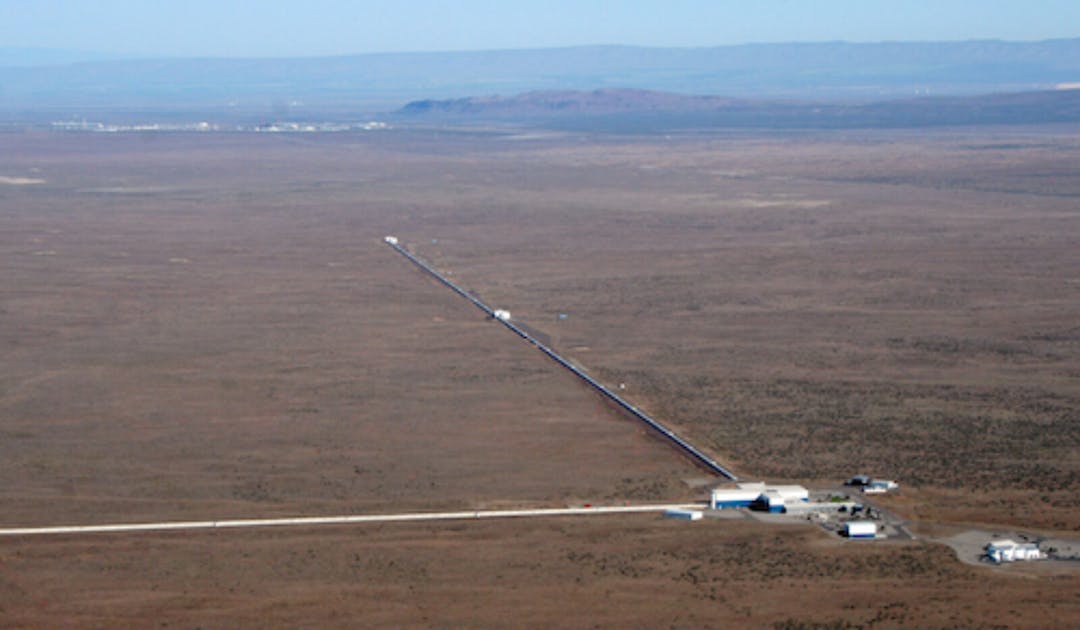
Merging neutron stars—the tiny, compact remnants of colossal stars—also share this knack for cranking out gravitational waves. LIGO has witnessed a couple neutron star mergers so far, which have the added bonus of producing light during their smash-ups. Other observatories seize upon this light for precious clues about the extreme astrophysics in play during the major mergers.
The most hotly anticipated gravitational wave generator at the moment, though, is a black hole and neutron star merger. LIGO gathered tantalizing evidence of just such a cosmic collision back on April 26, 2019. Researchers continue to scrutinize the intriguing signal in an effort to nail down its origins. "We certainly do not have a solid detection of a neutron star–black hole merger," says Fritschel, but the jury remains out. Such a pairing would offer significant new insights into the natures of both extreme cosmic objects, as well as potentially yield radiation that other instruments might pick up to allow still-deeper investigation.
As a piece of science equipment, known as an interferometer, LIGO is staggering. Its twin observatories are L-shaped structures, with each arm of the 'L' running 4 kilometers (2.5 miles). A high-powered laser is beamed down an arm and bounced off mirrors back to its source. The two lasers overlap in such a way as to cancel each other out so that no light comes out of the experiment. If, however, something throws off the ultra-precise alignment of the lasers, an interference pattern is produced.
The setup is exquisitely sensitive and would be thrown horribly out of a whack just by the passing of a truck on a nearby road. But a series of vibration damping systems work to isolate LIGO from virtually all Earthly disturbances. LIGO is thus poised to "feel" the passing of gravitational waves clean through our planet, which warp the lengths of the observatories' arms ever so slightly. Overall, LIGO is so sensitive that it can register displacements as little as around ten attometers, which is a 10-19 of a meter, or on the order of a ten-thousandth of the diameter of a proton—in other words, no matter how you slice it, simply mindboggling.
Building a paradoxically giant interferometer for this infinitesimal gravitational wave-hunting task was the brainchild of Rainer Weiss, an emeritus professor at MKI, and Kip Thorne of Caltech. For their groundbreaking work in developing LIGO, these scientists shared the 2016 Kavli Prize in Astrophysics with colleague Ronald Drever, also of Caltech.
LIGO's third observing run will wrap up next spring. That leaves plenty of time still to ring in new and powerful gravitational wave outpourings, especially from relatively close-by mergers of hefty objects. These hoped-for happenings would shower scientists with data to stringently test out our theories of the universe's workings.
"I am looking forward to detecting some more nearby events than we have seen so far," says Fritschel. "The signal-to-noise ratios would be significantly higher since we have improved the detectors, and this would allow us to extract more physics.