Liquid Fuels from Sunlight
by Alan S. Brown
Led by Caltech’s Harry Atwater, the Liquid Sunlight Alliance hopes to turn sunlight into gasoline and other fuels
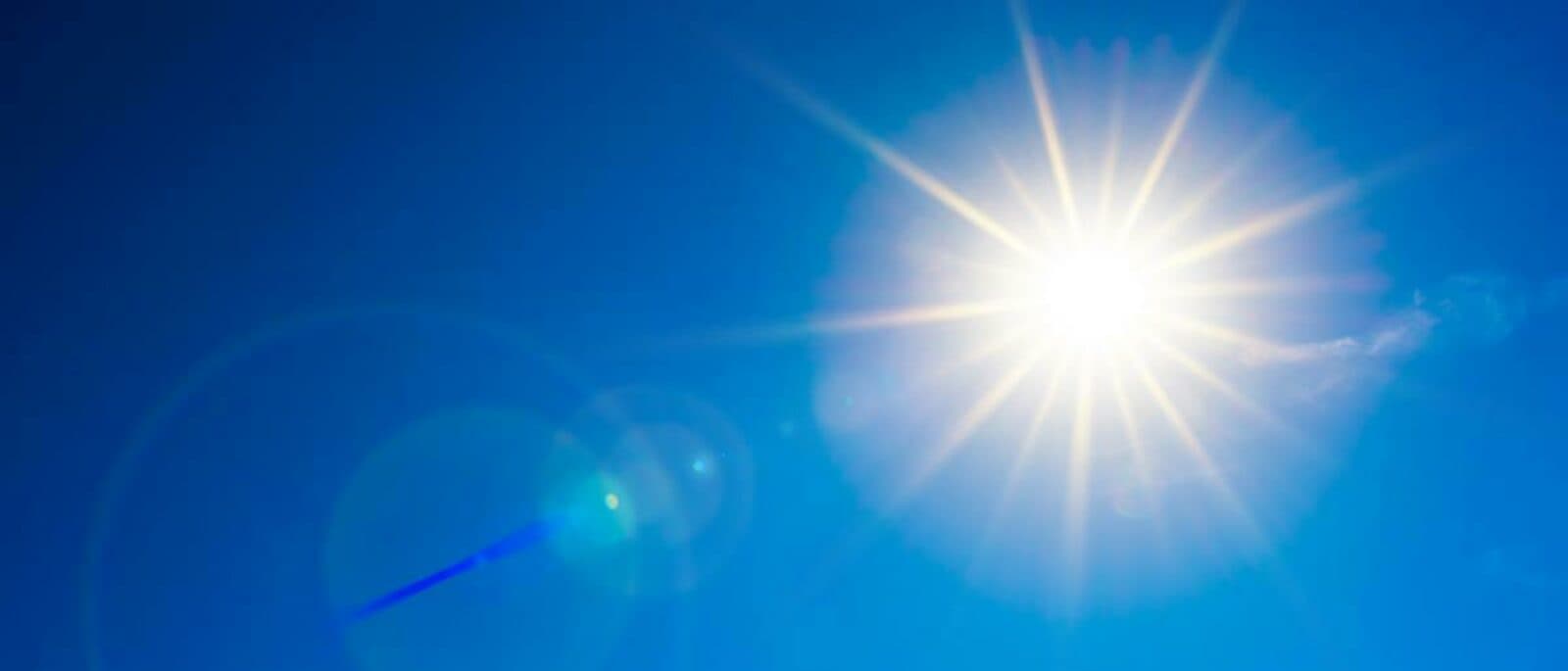
The Author
The Researcher
Over the past 15 years, solar energy has gone from a high-priced novelty to a technology that can generate power for less than oil and gas over the lifetime of a new project. Now researchers want to take solar one step further and use it to produce liquid fuels—sustainable, storable, and portable sources of energy.
This is exactly what nature does with photosynthesis. Green leaves transform water and carbon dioxide into the sugars that keep plants alive.
The new Liquid Sunlight Alliance, a consortium of research organizations led by Harry Atwater, a member of the Kavli Nanoscience Institute at California Institute of Technology, plans to use the same strategy to make gasoline, diesel, jet fuel, and other liquid fuels directly from the sun.
With solar electricity and batteries ascendent, why bother? One reason is that liquid fuels are a great way to store energy. Batteries, Atwater explained, work best storing charges for hours or up to a week or two. Liquid fuels, on the other hand, last for months to years, and are easily stored and delivered through existing infrastructure. Because they pack so much energy in a small volume, they are likely to continue finding uses for long trips where refueling is impractical, like international aviation, transoceanic shipping, and perhaps long-distance trucking.
Of course, burning liquid fuels emits carbon dioxide, a greenhouse gas, into the atmosphere. But since Atwater’s fuels will be made of atmospheric CO2, the only CO2 they will emit are molecules that were already in the atmosphere to begin with. CO2 used to make fuel and CO2 emissions will always remain in equilibrium.
Turning sunlight into liquid fuel—and perhaps, one day, pharmaceuticals and specialized chemicals—will require a new way of doing chemistry. Fortunately, the Liquid Sunlight Alliance have a plan for that.
Microenvironments
Atwater and his colleagues want to transform simple molecules, like CO2 and water (H2O), into fuel molecules. These consist of chains of up to 14 carbon atoms with their attached hydrogen atoms or hydroxyl (OH). To do this, they need catalysts, materials that lower the energy barrier needed for chemical reactions to occur.
Unfortunately, there is no way to go directly from simple molecules to complex multi-carbon chains. “If you want to go from carbon dioxide and water to methane [carbon surrounded by four hydrogens], you can imagine doing it in one step,” Atwater said. “But to go to something with lots of carbons, like butanol, there is no single catalytic transformation that anybody can envision to do that.”
Instead, Atwater envisions a series of cascading catalytic steps, each one adding a small segment until the entire molecule is complete. This is how photosynthesis builds sugar molecules: using enzymes to catalyze reactions in one chemically isolated microenvironment and passing the results to another for the next step.
The Liquid Sunlight Alliance’s predecessor, the Atwater-led Joint Center for Artificial Photosynthesis, demonstrated we can indeed build microenvironments using man-made catalysts. The team showed it could split water into hydrogen and oxygen and turn carbon dioxide into carbon monoxide.
Their new research endeavor will attempt to link these and other microenvironments in a single, integrated reactor. For that to work, each microenvironment’s catalyst must produce only the molecules they need without unwanted by-products that sabotage production. Those catalysts must also function under similar operating conditions, such as temperature and acidity, and last much longer than the few hundred hours previous systems have survived.
“Compared to what we did before, it’s like going from like an infield single to an over-the-fence home run,” Atwater said.
Rethinking Discovery
To hit it out of the ballpark, Atwater turned to computer modeling to screen large numbers of potential catalysts for the properties he needs.
“I’m an experimentalist by training, and regard theory [the basis for computer modeling] with mixture of enthusiasm and healthy skepticism,” he said. “But I’ve seen what’s happened in world of catalysis. There used to be a wide gap between theorists and experimentalists. The theorists could not make predictions that could be tested easily. But in the past five years, the gap’s been closing fast.”
Atwater’s models, which run on a large Department of Energy supercomputer, start with quantum mechanical calculations of how atoms and molecules accept, donate, and withhold electrons. This data feed into models that calculate the behavior and properties of potential catalysts.
Atwater is using computational models to screen tens of thousands of materials. With each iteration, the models make very slight changes in material composition and structure, then test for the combination of properties—from light absorption to durability—needed for each reaction. This takes place tens of thousands of times faster than trying to synthesize and test these materials in a lab.
It also enables the researchers to explore combinations of elements they might not have tested in the past. Most catalysts, for example, consist of only one or two metals or metal-oxygen combinations. Computer modeling, on the other hand, enables Atwater to search compositions with four, five, or six different elements.
“The number of possible compounds you could make with five or six elements is extremely large,” Atwater said. “Their complexity is way beyond the properties we could predict with deductive reasoning and intuition.”
His team is also using computational models to unravel the mechanisms by which new catalysts facilitate reactions in order to improve them.
Even so, Atwater needed to accelerate the discovery process. So he turned to co-design, a technique commonly used to develop complex industrial products and software. It involves setting guidelines early in the development process about how each subsystem will work with the others before beginning the project.
“This may be the first time anyone has used this approach in a basic science setting,” Atwater said. “Making multi-carbon products is so complex, you couldn’t have each team work on its own little area and then hope they would all fit together at the end. Since we couldn’t explore every path, we’re trying to do the fundamental science along a set of paths that are most likely to lead to that progress.”
The Liquid Sunlight Alliance has a $60 million grant for the next five years to show if its approach works. By 2025, Atwater hopes his team will have developed a couple of examples of connected microenvironments that go from carbon dioxide to multi-carbon liquid fuels.
“I don’t think we will have achieved the high efficiency or chemical purity that a commercial process demands,” he said. “But I hope we will have made the first steps along that road to multi-carbon products the people recognize as viable fuels.”