Mapping the Brain
Scientists at the Kavli Institute for Brain Science at Columbia University are working toward a real-time map of neural circuits in action.
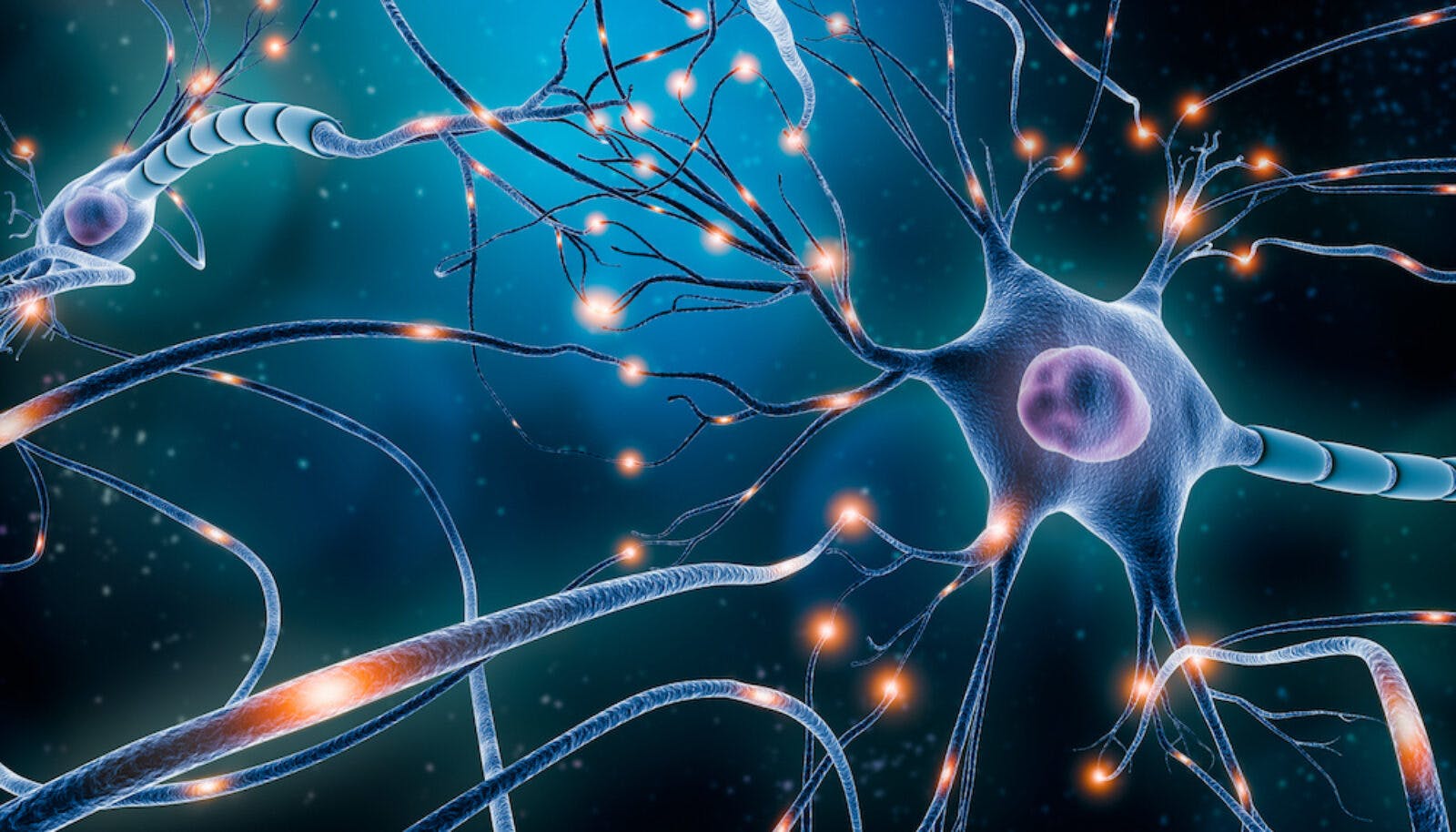
The Researchers
Neurobiologists have studied the brain for over a hundred years, yet they are still far from understanding its basic operations and the overall logic of its neural circuitry. Part of the problem is technical: Traditionally, scientists have studied the responses of neurons (brain cells) with electrodes, one neuron at a time. But the brain is composed of billions of neurons, anatomically arranged in precise circuits. The rules that govern how these neural circuits work is difficult to untangle unless one could actually record the activity of many neurons simultaneously – if possible in the living animal.
At the Kavli Institute for Brain Science at Columbia University Medical Center, researchers are developing novel imaging techniques to study this communication among brain cells. One of the Institute’s key goals, says its director Eric Kandel, is to “visualize the circuitry” of the brain. Toward that end, Kavli Columbia researchers are using advanced high-resolution microscopy to detect and record processes taking place on a molecular scale. They hope to understand the brain’s logic, the rules that make trillions of tiny electrochemical events work together to produce human thoughts, emotions and actions in all their complexity.
The Holy Grail of Neuroscience

Rafael Yuste, a neurobiologist and co-director of the Institute, says the “holy grail” of neuroscience is a “map of the electrical activity of the brain.” In essence, this would be a detailed, real-time motion picture of the circuits of the brain at work, built up from imagery of the electrical charges between neurons. To record those charges, he is using a method of microscopy based on high-intensity lasers to measure voltage across the 2-nanometer-wide membranes of dendritic spines, which protrude from neurons where the cells send and receive signals. This space is too small for traditional electrodes to probe, yet it’s where essentially all excitatory contacts in the brain take place. The laser beams home in on the interface between neural membranes and intercellular material. There, they touch off an amplified signal (at twice the energy of the incoming light) which is proportional to the voltage.
This new method, called second harmonic generation (SHG), developed in collaboration with Columbia Chemistry Professor Ken Eisenthal, is an advance on the older technique of calcium imaging (CI), also pioneered by Yuste, which records the electrical activity (firing) of neurons indirectly by detecting the calcium that enters them as they send or receive signals. Unlike CI, SHG is direct and instantaneous. It records the actual electrical activity of neurons, not just a chemical byproduct, and it shows the action in real time. With SHG, Yuste says, “we could get a handle on the interaction between neurons when they are actually talking to each other.”
Lighting Up Molecules
Another Kavli researcher, Steven Siegelbaum, uses a technology called two-photon microscopy to image pre-synaptic terminals (the nerve cell tips that send charges from one cell to another) within slices of living brain tissue. In this method, a very powerful laser beam strikes dye molecules at half the energy (twice the wavelength) needed to excite them. Outside a very narrow focal plane, nothing happens. Within that plane, photons are so concentrated that they interact with the dye in pairs, each of which has enough energy to light up the dye molecules. This “quantum trick,” as Siegelbaum calls it, enables researchers to make images at points deep inside a tissue sample without losing light to scattering or exciting dye at points other than the target.Siegelbaum’s research focus is on the hippocampus, a region that is crucial to memory in the mammalian brain. With two-photon microscopy and other techniques, he studies in detail how synaptic terminals change – creating memories and learning – when stimulated. Scientists know that a synapse strengthens, like a muscle, when it’s used to carry a signal. Siegelbaum and others are trying to find out exactly how this process works at the molecular level, and what roles are played by the cells on either side of the synapse, the “pre-synaptic” sender and the “post-synaptic” receiver. “There’s a big controversy as to whether the strengthening involves change in the pre-synaptic cell, or in the post-synaptic cell, or in some combination of the two,” he says.

Neurobiologist Craig Bailey uses confocal microscopy to track the learning-related changes in synapses of the sea slug Aplysia. This technology creates images by scanning objects with precisely focused beams of light. Following a path first charted by Kandel, whose pioneering studies with Aplysia helped earn him the 2000 Nobel Prize in Medicine or Physiology, Bailey studies how the animal’s neurons change with learning. In a classic experiment, scientists have shown how Aplysia loses its protective response when an experimenter repeatedly touches a sensitive spot – the tube with which it draws water into its gills. A key unanswered question is how the time span of this habituation – the long-term or short-term memory – is reflected in neuron structure. Bailey now uses imaging technology to observe the changes in the synaptic connections in single motor neurons, as they are subjected to physiological stimulation in a culture dish. “We are now able to monitor, in living synapses, in time-lapse imaging, changes at the molecular level that underlie the initiation as well as the stabilization of this neuron growth with learning and memory storage,” he says.
Nobel laureate Richard Axel is using two-photon microscopy and calcium imaging to determine how specific odorants are represented at various relay points in the olfactory system. What, he asks, is the higher logic of olfaction?
Thomas Jessell uses imaging to explore the neural circuitry in the spinal cord that controls and modulates movement through the environment.
Kandel is using special fluorescent markers that label messenger RNAs to track their movement from the cell body to the synapse in response to learning-related growth requirements.
The Brain's "Dark Matter"
All these scientists were investigating the brain long before Kavli Columbia started in 2004. But the establishment of the Institute gave new impetus to their work on neural circuits, including molecular manipulation, mathematical modeling and imaging. In imaging, the Institute advanced researcher’s capabilities with new technology, such as powerful laser microscopes. “There was a quantum improvement when [Fred] Kavli came on board,” Kandel says. Bailey says “the quality of the imaging we were getting was 100-fold less” before the new microscopes. Siegelbaum says the Institute is also drawing attention to the big picture – how all these neurons work together. “It has gotten all of us to focus on circuitry,” he says.
As images of the brain become sharper and closer to real time, some exciting scientific possibilities also become clearer. On the practical side, the “conversation” between neurons, as Siegelbaum calls it, may hold the key to curing diseases of memory such as Alzheimer’s. If scientists can understand just what causes synapses to strengthen as cells send their signals, he says, they “could rationally design drugs to combat memory loss.”
On a more philosophical plane, Yuste says the mapping of the brain’s electrical activity in real-time may help answer the long-standing question of the nature of the mind, a question hotly debated since Locke, Hume and Kant. Is the brain simply an organ for taking in sensory stimuli and turning them into behavior? Or does it have a built-in creativity, its own internal program, that generates mental states, thought and action? Yuste says the answer might lie in what he calls the brain’s “dark matter,” the neural activity that goes on constantly behind the activity evoked by sensory stimuli. Shining light into that realm may produce more than magnificent moving pictures. It could illuminate the brain’s own inherent logic – in effect, its mind.