Microbiomes and Machine Learning
by Alan S. Brown
By probing the human gut microbiome, Ilana Brito hopes to understand how tens of trillions of microbes interact to affect human health
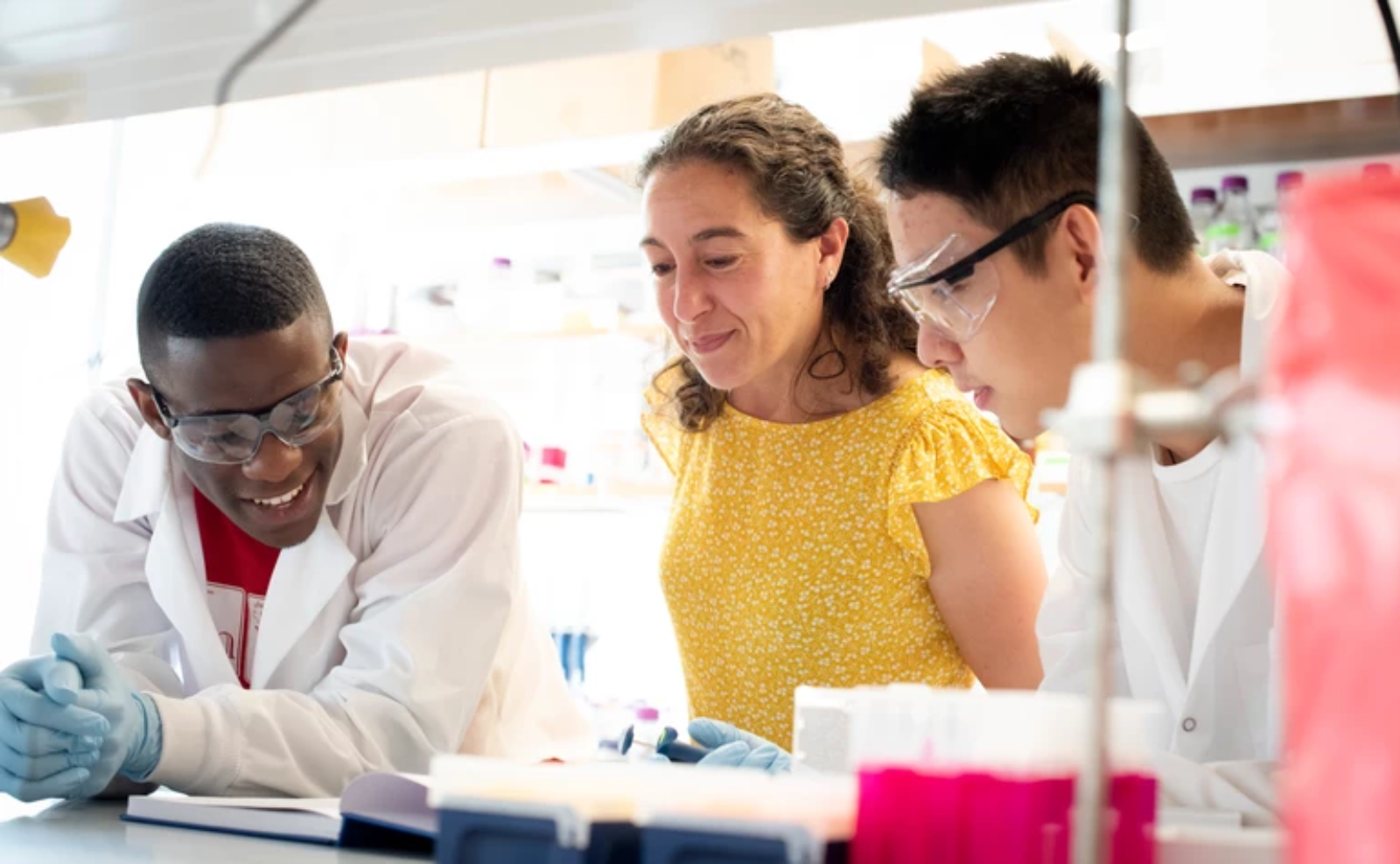
The Author
The Researcher
In 1900, one out of every three deaths in the United States were due to pneumonia, tuberculosis, diarrhea/enteritis, or diphtheria – all bacterial infections. Even something as innocuous as a paper cut, a scratch from a rose thorn, or a minor workplace accident could kill. Today, antibiotics make such outcomes unlikely. They also make possible chemotherapy, organ transplants, hip replacements, emergency room surgery, and a wealth of other medical miracles.
Imagining a world without antibiotics seems inconceivable. Yet, today, many strains have evolved to resist a broad range of antibiotics. This has occurred because bacteria that have survived antibiotics in the past can transfer their protective genes to bacteria with no resistance now.
This process is called horizontal gene transfer, and there is a great deal we do not know about it, said Ilana Brito, a professor of biomedical engineering and a member of the Kavli Institute at Cornell for Nanoscale Science. For example, do all bacteria transfer genes? Do they have favored partners? How do genes move between organisms? What triggers the transfer?
To answer those questions, Brito needed to explore the biological equivalent of an unexplored continent—the human gut biome, where tens of trillions of microorganisms live and interact with one another.
“The microbes there are altering our bodies and changing the effects of certain medical treatments,” she explained. “By bioprospecting in the human gut microbiome, we are trying to learn from these organisms. Maybe we can find new treatments for disease and better diagnostics. Perhaps the organisms themselves can act as treatments.”
Probing the biome
In biology, a biome is a large, self-sustaining ecosystem of plants, animals, and microscopic organisms. While not as large, the interactions among its thousands of distinct types of bacteria, fungi, viruses, and other one-celled organisms in the human gut biome are nearly as complex.
Most are known only by their genomes. Many remain unstudied because researchers cannot grow them in petri dishes. Yet this enormous community of microbes appear to play a role in everything from digestion to the immune system.
Brito believes the gut biome is ground zero for most horizontal gene transmission. Yet its sheer complexity, largely unknown composition, and the tiny scale of the interactions occurring within make it incredibly difficult to study.
Brito took a circuitous route to investigating the microbiome. She grew interested in infectious diseases as an undergraduate at Harvard University. After double-majoring in biology and government and doing field research on malaria in Mali for half a year, she returned to Boston to earn a Ph.D. in genetics at MIT.
Along the way, she became interested in the human microbiome. As a post-doctoral researcher, she went to the Fiji Islands to conduct one of the first large-scale studies of non-Western human microbiomes.
At the time, organisms within the microbiome community were identified by essentially shredding their genetic material, sequencing the pieces, and then trying to figure out which pieces went together. This provided some insight into the type of microbes that were present and some hints about their roles.
“If we saw a segment of DNA co-occurring with other pieces of DNA, we could assume they came from the same organism,” Brito said. “We would then start grouping these sequences together to create a genome.”
Using data from Fijian microbiomes, Brito used that approach to discover new organisms that had never been catalogued in Western public databases. The reassembled genomes told her something about the new types of bacteria she was discovering, but she wanted to know more.
Once she opened her own lab at Cornell, Brito began to apply a broad range of technologies—from growing mammalian tissues and isolating DNA, RNA, and proteins for analysis to genetic engineering, mouse models, and computational methods—to further her understanding of what she was finding.
Discoveries
These techniques gave Brito and other investigators new insights into the gut microbiome. For example, many gut bacteria live in colonies bound together in a biofilm held together by a mix of sugars, proteins, and DNA. So, where do they get the raw material to manufacture this structure? It turns out that some bacteria specialize in transforming gut secretions, like mucus, into structural materials.
Gut bacteria can also make proteins that act as drugs. There are a large but unknown number of these interactions, and perhaps a dozen of them have been well-studied, Brito said.
One example involves flagellin, the long protein that acts as a swishing tail that enables bacteria to swim. In mouse models, flagellin can interact with cell receptors and trigger processes that are involved in inflammation and perhaps diabetes. On the other hand, the shorter hairlike pilus proteins found on many bacteria can improve glucose tolerance in mice.
Using computer models, Brito’s group has sought to catalog these interactions. Some happen more in healthy subjects, others in people with diseases. Sometimes, the bacteria involved have proteins that resemble or are even identical to those found in disease pathogens.
She also sought to understand how bacteria exchange DNA and develop drug resistance in the gut microbiome. One mechanism involves plasmids. These are small, circular strands of DNA that can replicate independently of a cell’s chromosomes and move readily between bacteria. Should a plasmid acquire antibiotic resistance, it can impart that property to other bacteria that acquire it.
The same is true for transposons, segments of chromosomes that can change position within a bacterial genome or swap itself into the DNA of bacterial plasmids and phages (viruses that infect bacteria). Phages have the potential to spread antibiotic resistance through a bacterial community the way a cold circulates among humans.
Machine learning
Brito wants to predict which bacteria are most likely to transfer antibiotic-resistant genes to which pathogens. That would enable her to look at a patients’ microbiome and determine which antibiotics might work best on a given disease or the likelihood that a pathogen acquires immunity from a treatment.
Researchers know that not all bacteria choose to swap genes. Others that do add new genetic material may have preferred partners or undergo swaps only when they share specific environments with other bacteria. Some may interact only with plasmids, others with transposons.
The most common way to look for these gene transfer pathways is to isolate and test a single strain of bacteria with one or two other organisms at a time. This is like trying to assess a nation’s culture by looking at how a single family behaves at dinner—it provides hints but misses the complexity of a diverse reality.
Brito, on the other hand, hopes to capture some of those rich interactions by using techniques similar to those that helped her acquire data in Fiji. Using a technique called shotgun genome sequencing, she breaks organisms’ DNA into small fragments, then uses software to reassemble them into genomes.
At the same time, she uses related techniques to capture RNA transcripts, which identify which proteins the bacteria in the sample are building. This, in turn, tells her which cellular processes are active and which are not.
Ideally, this would enable the researchers to pull a string of data from their sample that ties everything together. All too often, however, the amount of data in that string forms a knot they cannot unravel.
The answer, Brito said, is machine learning. This is an artificial intelligence technique used to sort through data, make connections between that data, and then use what it has learned from the experience to improve its ability to dig deeper and find more meaningful connections.
The team used a variety of machine learning models to look at bacterial genomes with such functions as DNA replication and carbohydrate metabolism. The AI used this data to find signatures that indicated which bacteria were likely to swap genes and under what circumstances those exchanges occurred. This enabled her team to identify networks of bacteria likely to exchange genes and to predict possible genetic transfers that no one has observed yet.
What’s next?
“By looking at genomes with machine learning techniques, we can begin to answer our questions about microbial ecology and interactions,” Brito said. “Because machine learning can handle complexity really well, we can also think about using the same kind of approach in other spaces as well.
“We also want to refine our models further. We want to ask about whether a pathogen is acquiring or leaving behind drug-resistant plasmids. We want to create models that can help doctors pick the right drug to treat a particular pathogen, or maybe understand how to use antibiotics more safely in both medicine and farm animals,” she said.
Brito admits we have a long way to go to truly understand the human gut microbiome. Yet every advance we make brings us closer to stopping the rise of antibiotic-resistant bacteria, something our ancestors from 1900 would certainly approve of.