Nanoscale Windmills and More
by Alan S. Brown
Research highlights from Kavli Nanoscience Institutes
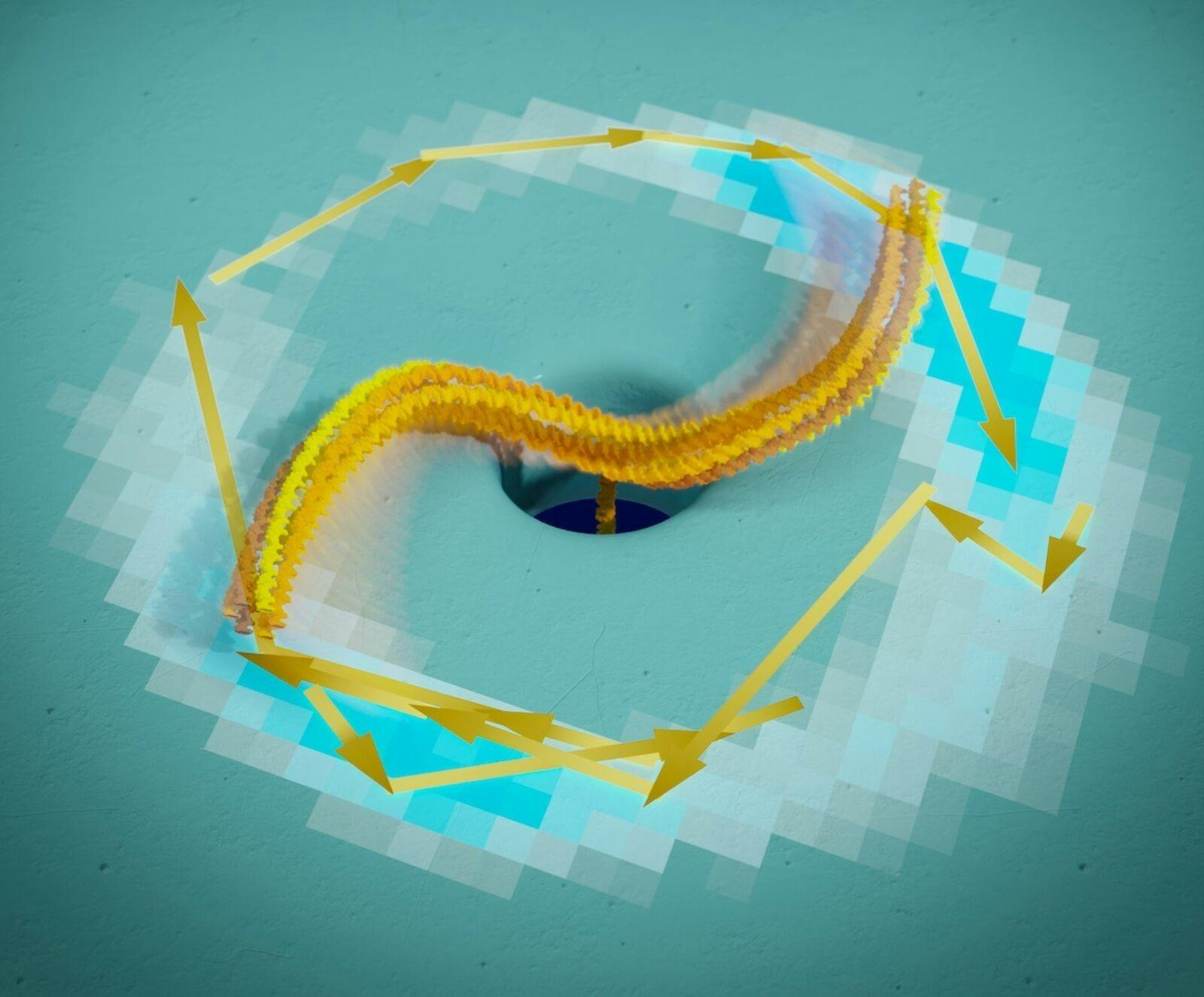
The Author
This month brings some fascinating research breakthroughs—and some practical advances on previous work. At the top of our list, a CRISPR-Cas system that cuts proteins rather than DNA or RNA and could have potential for medical treatments. Speaking of which, we see a significant advance in wearable sensors in terms of sensitivity and a breakthrough in rotating motors inside cells. Also, this month, we learn about faster cancer diagnosis in developing countries (as well as our own) and why the world’s largest Coca-Cola bottler is interested in a spinoff from a NASA competition.
CRISPR-Cas cuts proteins, not DNA
Researchers led by Stan Brouns have discovered a CRISPR-Cas system that slices proteins instead of DNA. Ordinarily, bacteria and archaea use CRISPR and Cas (CRISPR-associated protein) to cut the DNA (and sometimes RNA) of invading viruses, rendering them ineffective. Brouns, a member of the Kavli Institute for Nanoscience at Delft Technology University, has now discovered a CRISPR-Cas system that cuts a bacterium’s own proteins. Bacteria do this when a virus is overwhelming their defenses. Cutting proteins pushes the virus into dormancy, a type of hibernation, that starves the virus of energy and keeps it from replicating. Bacteria do this to prevent themselves from multiplying and infecting the bacteria around them. Right now, Brouns is interested in learning more about the new CRISPR-Cas system, which he hopes to modify to cut other proteins and perhaps use to sense bacterial infections.
Vastly improved wearable sensor
The stick-on wearable sensors developed by Kavli Nanoscience Institute at Caltech researcher Wei Gao faced a problem. The enzymes he uses to detect disease markers in sweat work only with a limited number of molecules. Antibodies could detect more molecules at lower concentrations, but they could only be used once. So, Gao came up with a clever workaround: molecularly imprinted polymers. They work much like a mold. If, for example, Gao wanted to detect the amino acid glutamine, he would create a polymer with glutamine molecules on the surface. Then he would chemically remove the glutamine, leaving behind the exact shape of the molecule he wanted to detect. When someone sweats onto the polymer, any glutamine in the fluid would get caught in the holes. To detect the molecules, Gao runs a current through the polymer. When the glutamine-shape holes are empty, the salty sweat makes it easy for the current to pass. As glutamine blocks the holes, the electrical resistance rises, signaling that glutamine is there. Gao also uses microfluidics to channel sweat to the sensor, so it works even when the subject is hardly sweating. He can also clean the sensors for reuse.
Nanoscale windmills in cells
Many biochemical reactions in cells have mechanical components. FoF1-ATP synthase, which makes fuel for cells, spins like a rotary motor. And now, Xin Shi, a postdoctoral researcher in the lab of Cees Dekker, a member and former director of the Kavli Institute of Nanotechnology at Delft Technical University, has found a way to make similar rotors from DNA. Shi observed a spinning 7-nanometer strand of synthetic DNA anchored to a nanopore, then worked with theorists to unravel what made this happen. They found that properly constructed DNA could harness energy from water and ion flow under an applied voltage or a difference in salt concentrations on the two sides of a membrane. Cells often use the latter to power processes like fuel synthesis and propulsion. The researchers then used a technique called DNA origami to design and build a self-organized 25-nanometer DNA rotor from the ground up, simply by applying an electric field. The rotors give researchers an entirely new way to create and power nanoscale applications at the cellular level.

Faster cancer diagnosis in Africa
The National Cancer Institute has awarded $4 million to expand testing of a device that can identify cases of Kaposi sarcoma in sub-Saharan Africa. The difficult-to-detect cancer attacks cells that line blood and lymph vessels and often signals the presence of an HIV infection. Physicians identify it with biopsies, but this takes days. In developing areas, patients may not wait that long even if the medical infrastructure was there to process biopsies. The KS-COMPLETE, developed by Kavli Institute at Cornell University engineer David Erickson and pathology professor Ethel Cesarman, returns a diagnosis in less than one hour. It has two components: the SLICER automatically processes biopsy samples into small microcores. They go into TINY (Tiny Isothermal Nucleic acid quantification sYstem), which identifies the presence of Kaposi sarcoma-associated herpesvirus by isolating its DNA and using solar heating to amplify the DNA to detectable amounts. Based on 506 biopsies from three HIV clinics in Uganda, TINY has achieved 97% sensitivity, 92% specificity and 96% accuracy. The grant will also enable Erickson to improve SLICER. He also believes the system could be used to identify other cancers and diseases.
Electrochemical reduction goes better with Coke!
Mars has lots of atmospheric CO2 and NASA wants to use it to make carbohydrates to feed astronauts and fuel bacteria in bioreactors producing chemicals, pharmaceuticals, and other useful products. So, NASA sponsored the CO2 Conversion Challenge to develop an inorganic route to converting CO2 to sugars. Last year, NASA announced that a team led by Peidong Yang, co-director of the Kavli Energy NanoScience Institute at UC Berkeley, was one of three challenge winners. This year, Coca-Cola Europacific Partners, the world’s largest Coca-Cola bottler, announced plans to partner with Yang to develop a scalable process to recycle CO2 into sugar for drinks and, eventually, biodegradable packaging materials. Yang’s technique uses electrochemical reduction with a catalyst to transform CO2 into glycolaldehyde and formaldehyde, which are then combined to form a variety of sugars. Scaling up would involve reducing the number of byproduct chemicals formed along the way and boosting the yield of desirable sugars. If the researchers can do this successfully, they would have a big impact on Coca-Cola’s carbon footprint, which is one-quarter agricultural products.