Nanoscience Goes the Distance
by Alan S. Brown
Telemedicine, quantum magnets, and more from the Kavli Nanoscience Institutes
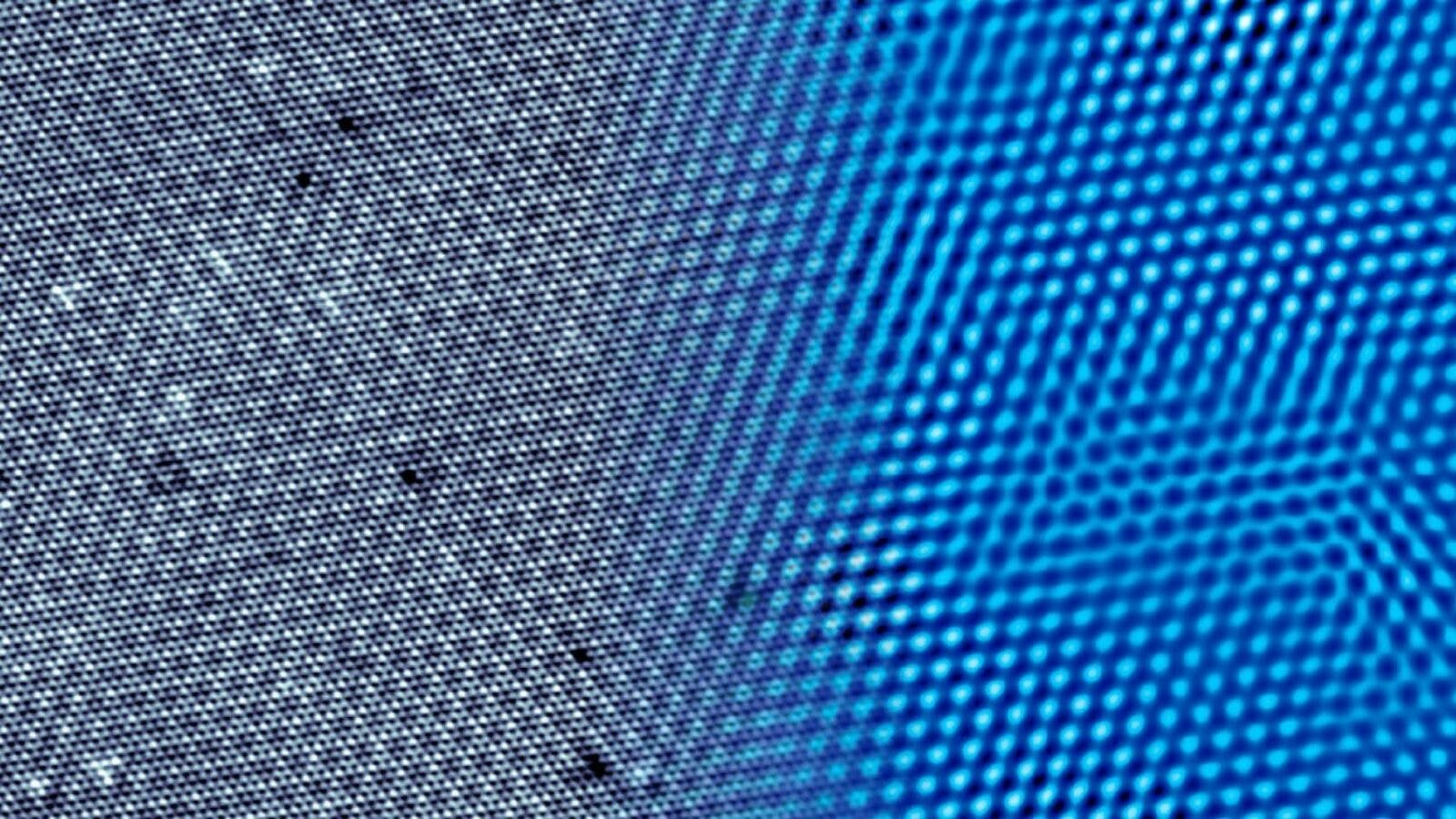
The Author
The summer is a great time to get outdoors, and many weekend athletes track their health stats with ubiquitous Fitbits, Apple watches, and other devices. This is only the beginning of the move to connected medicine, which certainly picked up steam during the COVID pandemic when many people communicated with their doctors online. Now, the National Science Foundation is launching a research group to move this area forward and Caltech nano researchers are heavily involved. Also on the health front this month, you can find out about how differences in gut microbiomes might lead to new therapies. And we look at a new way of growing nanoplastic composite crystals, quantum magnets, and insights into superconductivity.
Streaming healthcare data
If you already wear a Fitbit or Apple watches that continually captures health data, you’ve already taken a step into the future of medical care. Portable healthcare monitors are becoming more common and may one day become an essential tool in connecting people with doctors. That is the rationale behind the National Science Foundation’s $3 million investment in the new Center to Stream Healthcare in Place, whose members include California Institute of Technology, University of Arizona, Baylor College of Medicine, and University of Southern California. Additional funding will come from private companies that want to join the group. At Caltech, the effort is being led by Kavli Nanoscience Institute member Chiara Daraio, whose goal is to go from benchtop to bedside. The researchers, who include Kavli members Axel Scherer and Wei Gao, will focus on the wearable, portable or implantable sensors and AI tools for data analysis and visualization that will make remote medicine possible.
Where you live determines your gut microbiome
The human gut microbiome—several pounds worth of multitudinous species bacteria, viruses, protozoa, and fungi—continues to surprise. The right mix can boost immune response and the wrong mix may be responsible for a variety of diseases. Now, a team led by Kavli Institute at Cornell for Nanoscale Science member Ilana Brito has found that microbiomes vary by geography. The differences could be due to everything from genetics and diet to antibiotic use, sanitation, and exposure to infections. Brito worked with germ-free mice and “humanized” them with gut bacteria from people from the United States, Guatemala, and Fiji. She found some microbiomes were better at resisting certain diseases, and that mice that lived together picked up resistance from those with the strongest microbiomes. The ability to transfer resistance to infection shows the microbiome’s potential for therapeutic treatments.
Nanoplastic composites put themselves together
Getting crystals to self-assemble at the nanoscale has led to some amazing breakthroughs like the quantum dots of pure color in QLED television displays and peptoid artificial proteins. Now, a team lead by Ting Xu, a member of the Kavli Energy NanoScience Institute at Berkeley, has found a way to create self-assembling nanocrystals of common plastics and metal nanoparticles. The process is blazingly fast — it takes minutes rather than hours or days — and uses common plastics. Like so many surprising discoveries, it happened by accident. Xu’s doctoral student, Yiwen Qian, left a solution of gold nanoparticles grafted to polystyrene in toluene in the lab for a few days. When she analyzed it, it had formed a crystal much faster than expected. It turns out that a small amount of the container’s polyolefin polymer liner had leached into the mix, and it made all the difference in speed. Xu and Qian are now learning to control the gold-plastic nanocomposite and want to see if they can use it to make a transistor. Ultimately, this could prove to be a way to build nanoscale electronics from the ground up.
Taming quantum magnets
Building materials at nanoscale dimensions enables researchers to create things that could not be made any other way. One of them is van der Waals magnets. These consist of stacks of atomically thin (2D) materials bound to each other in the third dimension by weak van der Waals forces. Theorists think they could be used for next-generation data storage or perhaps spintronics-based quantum computing. Unfortunately, their magnetic order collapses as temperatures begin to rise above absolute zero. The theoretical solution is to pin the material’s magnetic spins in preferred orientations, but how? Now a team led by Andrea Caviglia, a member of the Kavli Institute of Nanoscience Delft, has figured out how to do this. He uses picosecond blasts of light at energetic enough wavelengths to change the orbital state of the material’s electrons. This works for as long as the light is shining, but they researchers believe they may be able to extend it with longer pulses.
More quantum materials than you might think
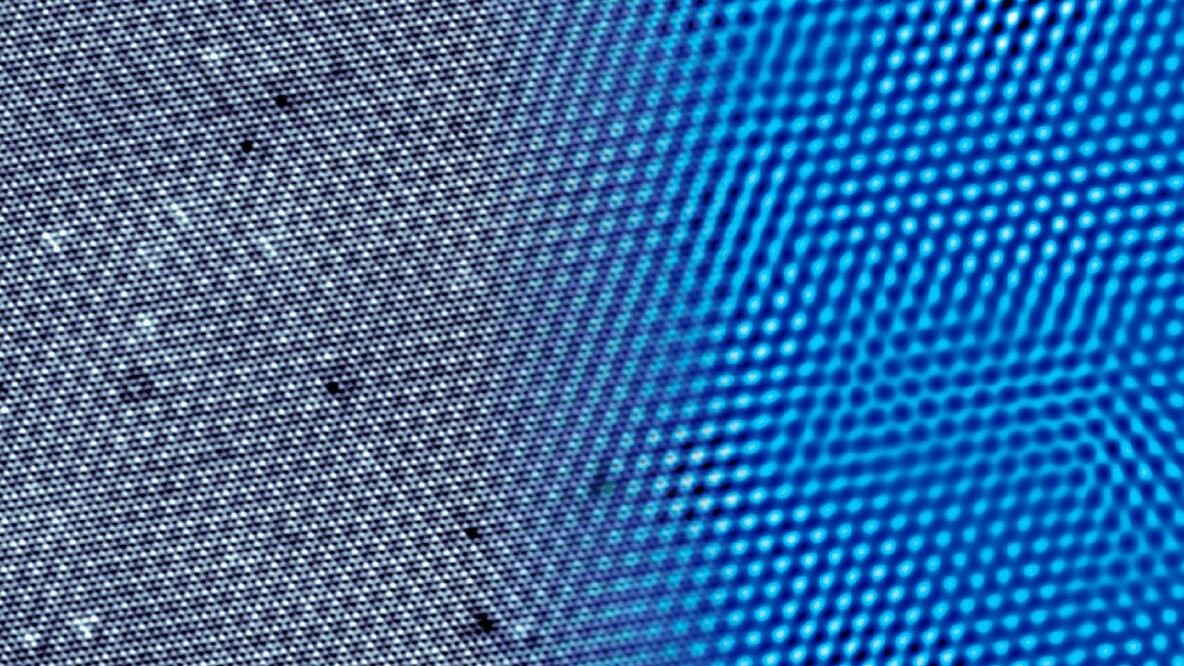
Five years ago, after more than 50 years of searching, Kavli Institute at Cornell physicist J.C. Séamus Davis discovered something called a Cooper pair density wave in copper oxides laced with other metals. Now, by upping the spatial resolution of his highly specialized scanning Josephson tunneling microscope, he has identified the same phenomena in niobium dichalcogenide, a member of the large and technologically important transition metal dichalcogenide family. Cooper pairs are electrons that share a single quantum state and carry electrical current without any resistance and are found in superconductors. When they vary in density over an area, they form Cooper waves. Understanding the difference between the two states might enable researchers to design superconductors that work at warmer temperatures.