Nanoscience that Stops a Speeding Bullet
by Alan S. Brown
A nano-architected material more effective at stopping a projectile than Kevlar, and other highlights from Kavli Institutes in Nanoscience
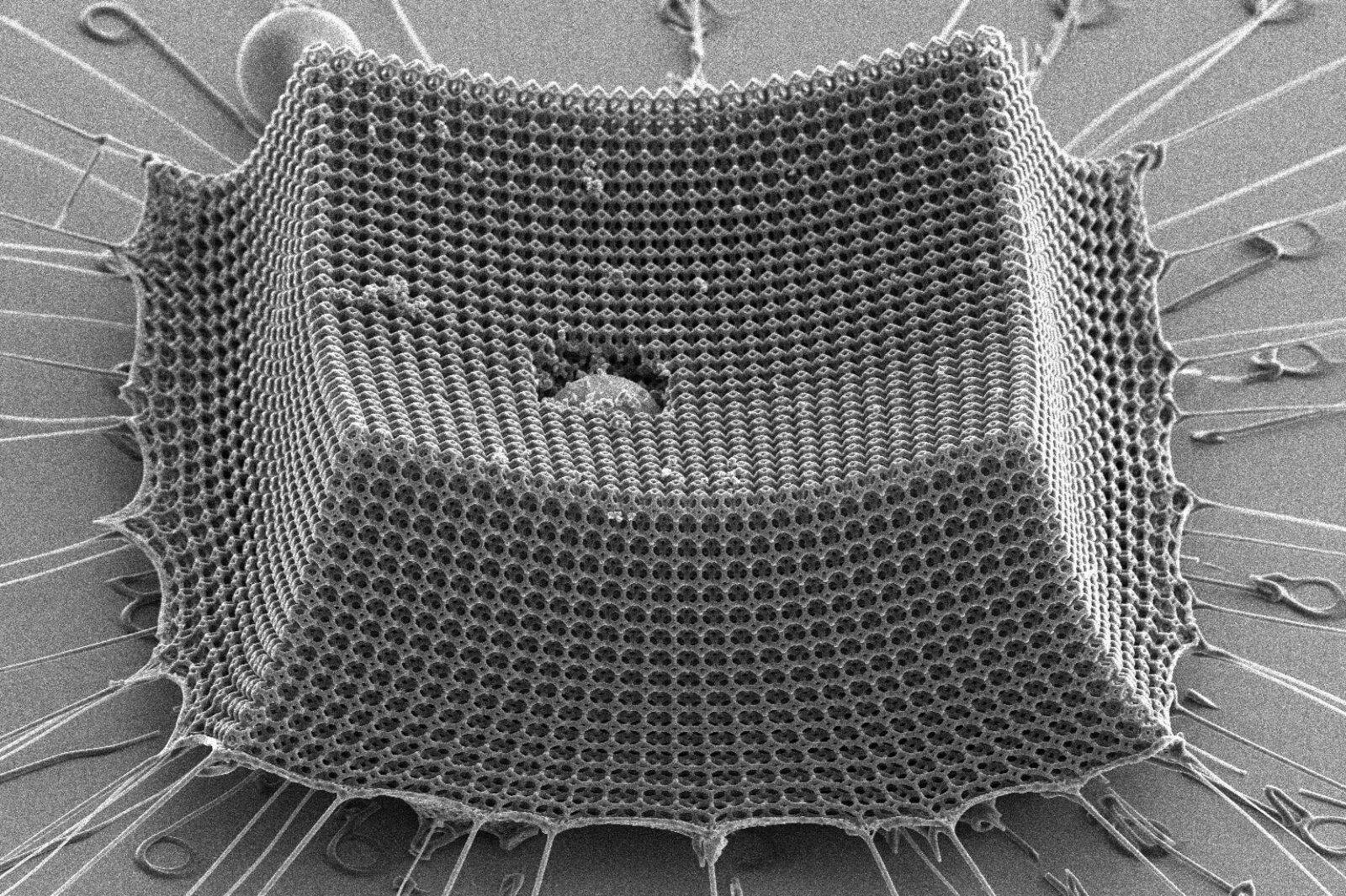
The Author
As summer warms up, so does the potential of nanoscience. We’ll start with one that is topical: with record heat roasting the United States and other parts of the world, many are realizing we need to grow more drought-resistant plants. To do that genetically, we need more detailed information on how plants handle water. A new aerogel nanosensor could provide that form the interior of a leaf. We will also look at a powerful but small device to probe magnetic materials, what graphene can tell us abut our heart, how nano-architected materials can stop a speeding nano-bullet, and new superconductivity secrets.
Nanosensors breed better plants
How do you breed more drought-resistant crops? One way is to understand how different plant varieties manage water within their leaves. Until now, that requires either disrupting leaf function or chopping up the leaf to sample it. Now, a team led by Abraham Stroock, a member of the Kavli Institute at Cornell, and graduate student Piyush Jain, has found a way to do it with a minimally invasive nanoscale reporter. The technique involves injecting nanoparticles made from a soft synthetic hydrogel (called AquaDust), which swells and shrinks depending on water availability in the leaf. It also contains dyes that fluoresce, depending on how close they are to other AquaDust particles. The more they swell, the closer they grow. This lets the researchers look at how water waxes and wanes within an individual leaf, helping researchers build more water-efficient crops.
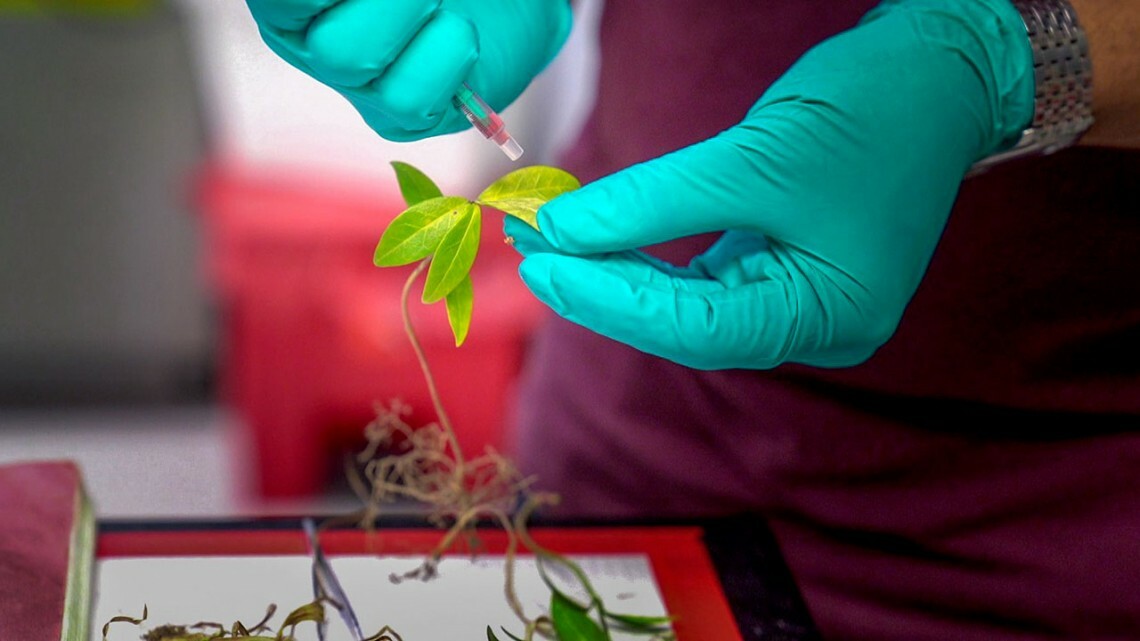
A synchrotron on your desktop?
Synchrotrons are football-sized electron accelerators that produce intense beams of light that enable scientists to look at samples with extremely high spatial and time resolution. That’s why researchers are always fighting to get research time on them. Now, Kavli Institute at Cornell member Greg Fuchs and his postdoctoral researcher Chi Zhang have developed a small system that provides similar nanoscale and picoscale resolution without trading one for the other. And, most amazingly, the new magneto-thermal imaging technology is small enough to fit on a lab bench. It works by focusing a laser onto a scanning probe, applying heat to a microscopic swath of a sample, and measuring the resulting electrical voltage for local magnetic information. The system is designed to work with magnetic materials and could make it easier for researchers to investigate quantum magnetic spins at the nanoscale.
Graphene images heartbeats
Medical researchers want to know more about how networks of cells control the beating of our hearts using electrical pulses. The problem is that they are limited by measurement techniques. These usually involve electrodes or chemical dyes, but they only return information for the point where they are used. What if we could measure how voltages move across a broader swatch of heart tissue? Using a graphene sheet to continuously monitor electrical activity over all the tissues it touches might enable them to do just that. This would let researchers image how electrical pulses move through neural networks with a diversity of cells that control the contractions of the heart. The technique also works with conventional microscopy, so it is possible to dye specific cells, then use graphene to measure how electrical currents flow between them. The technology was developed by a team led by Feng Wang, a member of the Kavli Energy NanoScience Institute at Berkeley.
Nanostructure that acts like Kevlar
Kevlar is the standard for bullet-proof vests because of its ability to stop a speeding projectile by bending rather than breaking. Now, Julia Greer, director of the Kavli Nanoscience Institute at Caltech, and researchers at ETH Zurich, have developed a nano-architected material that is, pound for pound, more effective at stopping a projectile than Kevlar. Thinner than a human hair, the material is made of interconnected tetrakaidecahedrons (six faces with four sides, eight faces with eight sides) made of carbon struts formed under extreme heat. At the nanoscale, carbon is less brittle than larger samples. The tetrakaidecahedrons take advantage of that, creating a rubbery, bending-dominated architecture. This is good enough to withstand the ultrasonic impact of a microparticle fired at it. If researchers could find a way to scale up production, the material could be used for armor, shields, and protective coatings.
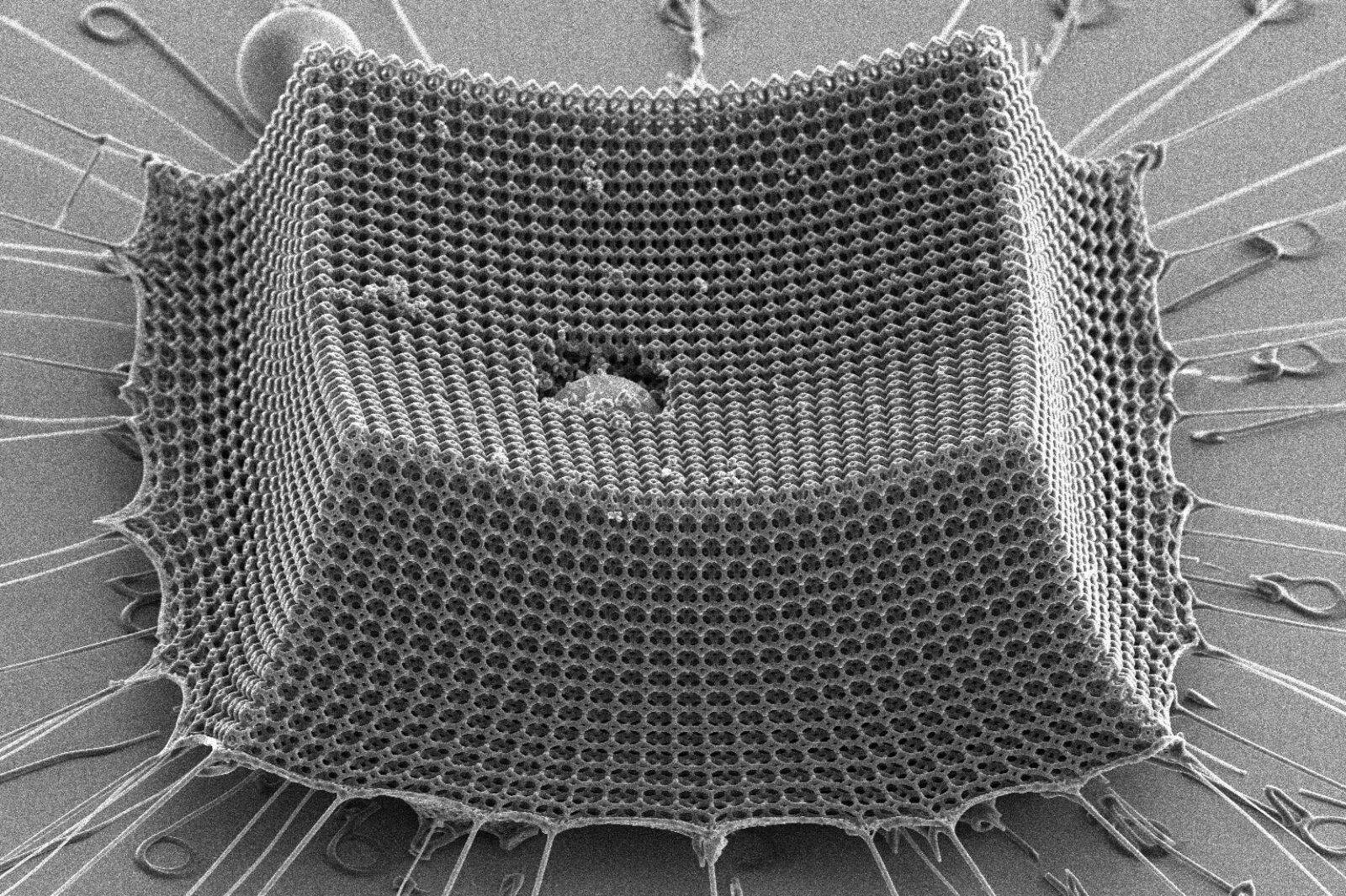
Superconductor secrets
Since the discovery of high-temperature superconductors—materials that deliver electrical current without any resistance—35 years ago, researchers have been looking for ways to keep them operating at room temperatures. The payoff would dramatically transform power grid efficiency. Now, a team led by Kavli Institute at Cornell member Kyle Shen has unearthed some interesting insights into iron selenide. This material turns superconducting at 8 kelvin, but turn it into a monolayer film and its critical temperature jumps to 30 or 60 kelvin. Usually, making materials thinner savages their superconducting properties. To study this fragile film, which disintegrates in air, Shen’s team built a suite of vacuum chambers to conduct research. They now have some idea why the temperature rises (it has to do with electron pairing) and why researchers see multiple superconducting temperatures. Next, they plan to use what they have learned to boost its operating temperature.