Diseases In A Dish: Modeling Mental Disorders
Using skin cells from patients with mental disorders, scientists are creating brain cells that are now providing extraordinary insights into afflictions like schizophrenia and Parkinson’s disease.
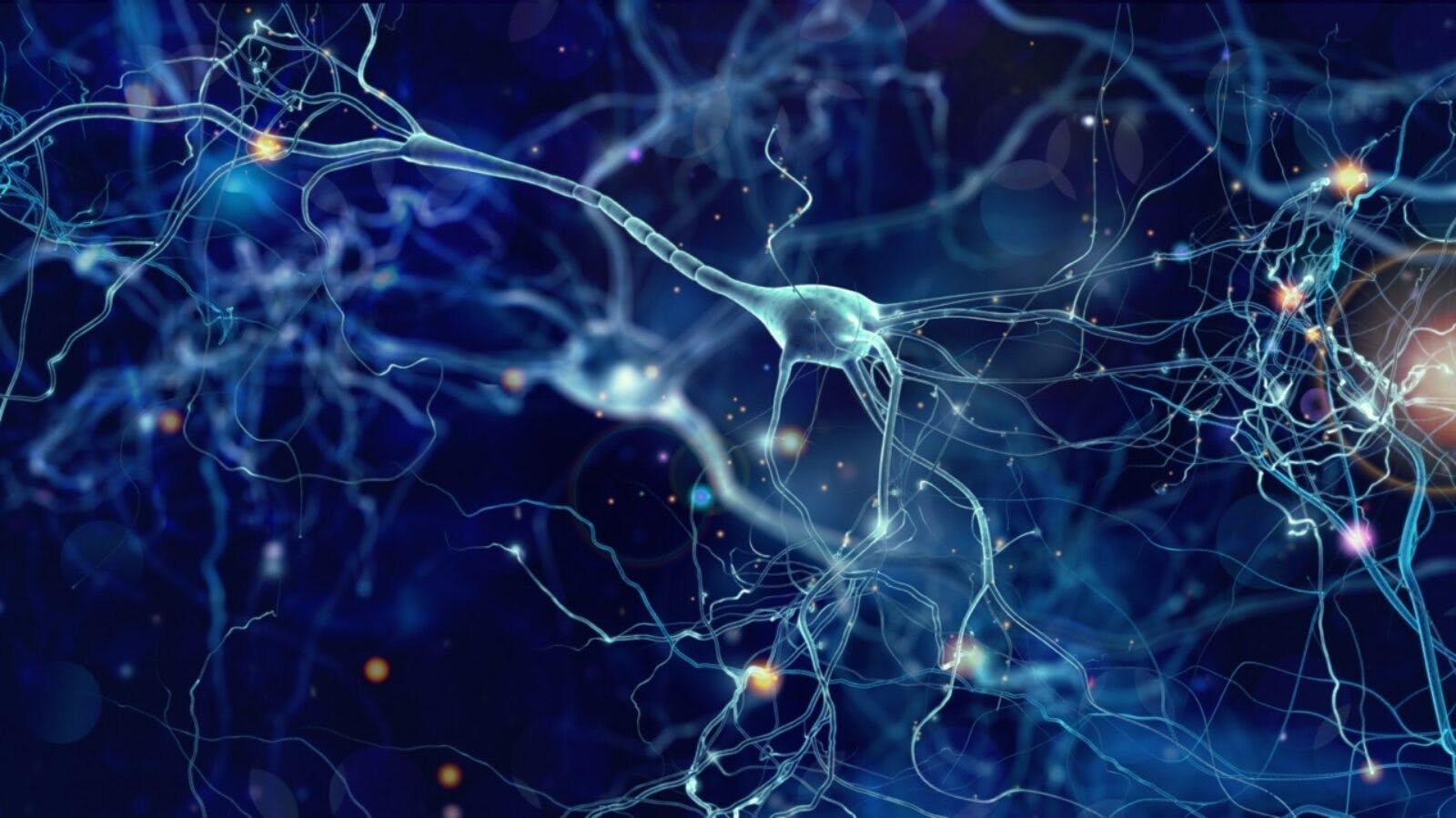
The Researchers
For many poorly understood mental disorders, such as schizophrenia or autism, scientists often wish they could turn back the clock to uncover what has gone wrong in the brains of these patients, and how to right it before much brain damage ensues. But now, thanks to recent developments in the lab, that wish is coming true.

Researchers are using genetic engineering and growth factors to reprogram the skin cells of patients with schizophrenia, autism, and other neurological disorders and grow them into brain cells in the laboratory. There, under their careful watch, investigators can detect inherent defects in how neurons develop or function, or see what environmental toxins or other factors prod them to misbehave in the petri dish. With these “diseases in a dish” they can also test the effectiveness of drugs that can right missteps in development, or counter the harm of environmental insults.
“It’s quite amazing that we can recapitulate a psychiatric disease in a petri dish,” says neuroscientist Fred (Rusty) Gage, a professor of genetics at the Salk Institute for Biological Studies and member of the executive committee of the Kavli Institute for Brain and Mind (KIBM) at the University of California, San Diego. “This allows us to identify subtle changes in the functioning of neuronal circuits that we never had access to before.”
Below is an edited transcript of a conversation with Gage and Anirvan Ghosh, a neurobiologist at the University of California, San Diego and also an executive committee member of KIBM. Both researchers are on the cutting edge of disease-in-a-dish modeling of neurological disorders. Gage and Ghosh discuss how human skin cells induced to return to an immature state (“induced pluripotent stem cells” or IPS cells) are revolutionizing our understanding and treatment of mental and neurodegenerative disorders, such as Parkinson’s disease, as well as leading to new models of drug development for all diseases.
THE KAVLI FOUNDATION (TKF): Dr. Gage, in your model you found that neurons look pretty similar between schizophrenic patients and normal controls, and it is just the connections between them (synapses) that are different, right?
FRED "RUSTY" GAGE: Yes. That doesn’t mean on closer inspection, and with better tools, more profound or subtle changes won’t be found. I wouldn’t be surprised if some more specific defects are revealed by a more sophisticated tool.
GAGE: We have to admit to ourselves this is a model of what might be going on in the brain and not think of it as a one-to-one relationship. It’s too soon to ask if this is how it happens in a patient; instead we are asking how do these drugs affect neurons.
TKF: So what are some of the surprises this modeling has revealed so far?
GAGE: One surprise is that neurons appear to undergo structural changes when they are given neuropsychiatric drugs. This is unexpected, as since the 1970’s companies have developed neuropsychiatric drugs on the premise that you modulate mood by regulating the amount of chemical signals available in the brain. These chemical signals are called neurotransmitters, and consequently the drugs have focused on modulating neurotransmitters such as dopamine and serotonin.
But one of the take home messages I got from my study is it’s not just the moment-to-moment regulation of dopamine that may be affecting the symptoms of schizophrenia, but the structural organization of how these synapses interact with each other. In other words, changing the regulation of dopamine or some other compound appears to have the additional effect of causing structural modifications that also affect how neurons interact.
TKF: This might explain why many of these drugs require a long period of time before a patient experiences a benefit.
GAGE: Exactly. If depression is merely due to a modulation of transmitter content and its receptor affinity, why wouldn’t these anti-depressants have their effects immediately? Normally it takes weeks. One of the emerging ideas is that part of the reason it takes longer is these drugs have other effects than what we’ve anticipated. Our findings support that possibility. Looking ahead, the next generation of drugs may not target dopamine or serotonin concentrations but instead the structure and function of synapses.
TKF: Dr. Ghosh, what other surprises has this modeling revealed?
ANIRVAN GHOSH: I think it’s remarkable what Rusty uncovered about schizophrenia. This is a pretty broad disorder and one we've suspected may have many different genetic causes. Yet they found there were shared cellular traits (phenotype) between the patients. This is a very exciting result because it raises the possibility of being able to find the phenotype—physical hallmarks--that might be shared for most, if not all, individuals with the disease.
TKF: What technological advances are needed to explore this further?
GAGE: One limitation is we haven’t differentiated the cells into specific cell types—neuronal subtypes. Right now we’re just laying these neurons down and allowing them to form connections as they might. Looking ahead, it’s going to be important for us to differentiate the cells. For example, to differentiate and model the cortical neurons, which are responsible for thinking tasks, or the hippocampal neurons, which are responsible for memory tasks. I can one day see us using microfluidic chambers to achieve this. They will allow us to compartmentalize microscopically specific subtypes of neurons in certain locations, and then regulate how they connect to each other. That way you can simulate in a more accurate manner how these subtypes connect with each other in the brain. The future of this is really exciting because the dish is going to get much more complicated.
TKF: So you’ll be able to combine the "disease in a dish" with a "lab on a chip"?
GAGE: Yes, the bioengineering part of this is becoming very exciting and interesting, and a lot of us will be relying heavily on that. It will enable three-dimensional cultures so you can have dopamine neurons projecting through a gradient into the types of neurons affected by Parkinson’s disease, for example. This way you can set up a whole neuronal network or circuitry that hopefully will be akin to what you see in the brain. We’re also trying to figure out what role inflammation plays in Parkinson’s disease. So for our model of this disease, which we are currently developing, we have to generate a variety of other brain cell types besides neurons that are thought to foster immune responses in the brain.
TKF: And you can compare mental disorders literally side-by-side; or rather, dish-by-dish.
GAGE: That’s the idea. As we accumulate models for these diseases — bipolar disease, schizophrenia, depression, autism — we are going to be able to explore if there are really differences between them that exist on a cellular or gene expression level.

GHOSH: I’m also really excited about using this platform to stratify patient groups and develop therapies that are more appropriate for them. For example, there are many causes for autism, so the same drug may not work for all patients with this disorder. But we could see how the cultured neurons of these patients respond to a particular drug or stimulus. Based on that, we could then classify them into specific groups and thereby provide a treatment that would be more effective for them. One can imagine down the road in a couple of years, if you have a child with autism, you could use this kind of platform to determine what kind of subtype he falls into, which would influence what kind of treatment he’ll get.
GAGE: I agree. There is going to be an exciting interface between basic scientists and clinicians. In schizophrenia, for example, it’s not unusual for a physician to test three or four different psychoactive drugs before they find the one a patient can minimally respond to. But suppose we could stratify patients to select the most likely appropriate drug based on their own brain cells and how they function? Furthermore, this will help us understand mechanistically how and why some patients may respond to the same drugs and others do not.
TKF: It seems the use of more selective and targeted drugs could have a revolutionary effect on the drug industry. What sense do you have of the support by drug manufacturers for this type of modeling?
GHOSH: There’s a lot of interest in using this as a platform for drug screens and drug discovery, and not necessarily waiting for five years to see how it develops. This is pretty surprising and quite bold, because many of the papers that report differences are incredibly recent and haven’t been reported in multiple labs. Normally industry tends to be pretty conservative about getting into these sorts of things. In the past, when we’ve talked to various pharmaceutical companies about one program or another in our lab, they often want to wait until it’s been developed into a preliminary screen, and would rather work with biotech companies for collaborations—they’ve often been the intermediate window before a pharmaceutical company would step in. But in this case, Roche and I have been active in directly interacting with various academic groups.
GAGE: I see it too. The pharmaceutical industry is really getting interested and supporting more interactions between basic laboratories and their own work. There is a tighter link between the basic science and the clinical science labs. We talk to these guys all the time now about the patients, which is really amazing. By working with clinicians caring for patients, we basic scientists are gaining a lot of inside information. The clinicians are also benefiting and now more and more clinicians are calling up and saying, ‘We’ve got this really interesting group of patients, and we’d love to see whether or not you could do something with them.’ We’re beginning to formulate specific hypotheses about what might be there, given what we know now. It’s striking that we’re already on the bridge between the lab and the clinic. It’s also exciting to see the young people coming into the field. These people, who are trained in cell biology, molecular biology, basic physiology or in other specialties, are now able to contribute to specific disease-related studies in a really viable way.
TKF: How is this new dynamic being fostered?
GHOSH: The interactions between basic science labs, industry and patient foundations are changing and proving very productive, and from the beginning, the California Institute for Regenerative Medicine has had a huge influence. It’s getting many people into this area and exploring things that otherwise they wouldn’t have, our lab included. The foundations – which are often associated with specific diseases –have also played a very positive role in bringing together all these different groups and getting them to work effectively together. In fact, the patient foundations are incredibly important because they are the most effective in making the case for supporting this research at places like NIH and Congress.
Thanks to all of this, today there is more talk about how one goes from basic science observations to potential therapies. It’s an unusual kind of alliance that has evolved out of this human stem cell work and does not exist so much in other areas of biology. It’s a new and really exciting model to get scientists to work with groups that are involved in drug development.
TKF: What sorts of more basic insights is this modeling providing?
GHOSH: We’re learning a lot about development of normal human neurons, which would have been impossible previously, so there’s a deep knowledge base in terms of understanding how cells mature and differentiate, how synapses behave, and perhaps circuits involving neurons behave. This is going to lead to a deep baseline understanding of various disorders. There also might be certain disorders you can treat by transplanting a small number of differentiated cells, so this knowledge would be useful for that. Understanding the pathways that allow the cells to divide or proliferate could also be used to manipulate cells in a particular aspect of the nervous system. There are populations of cells that divide in the mature brain, and the knowledge we gain from the in vitro studies could help influence how one might be able to manipulate and use that newly generated population of cells in the brain in more therapeutic ways. Those haven’t been explored much, but could be useful in the future.
GAGE: I think it will also give us insights into issues related to evolution. We basically have an entire zoo of neurons in a dish now, so with these models we can begin to look at underlying mechanisms that may be different between neurons of different species, and explore what is the basis of these differences between species. We can even look at our closer relatives and get to the essence of what makes us human.