The Next Life of Silicon
by Alan S. Brown
Perhaps the most amazing thing about silicon-based technology is that each new generation of processors delivers more power for less cost.
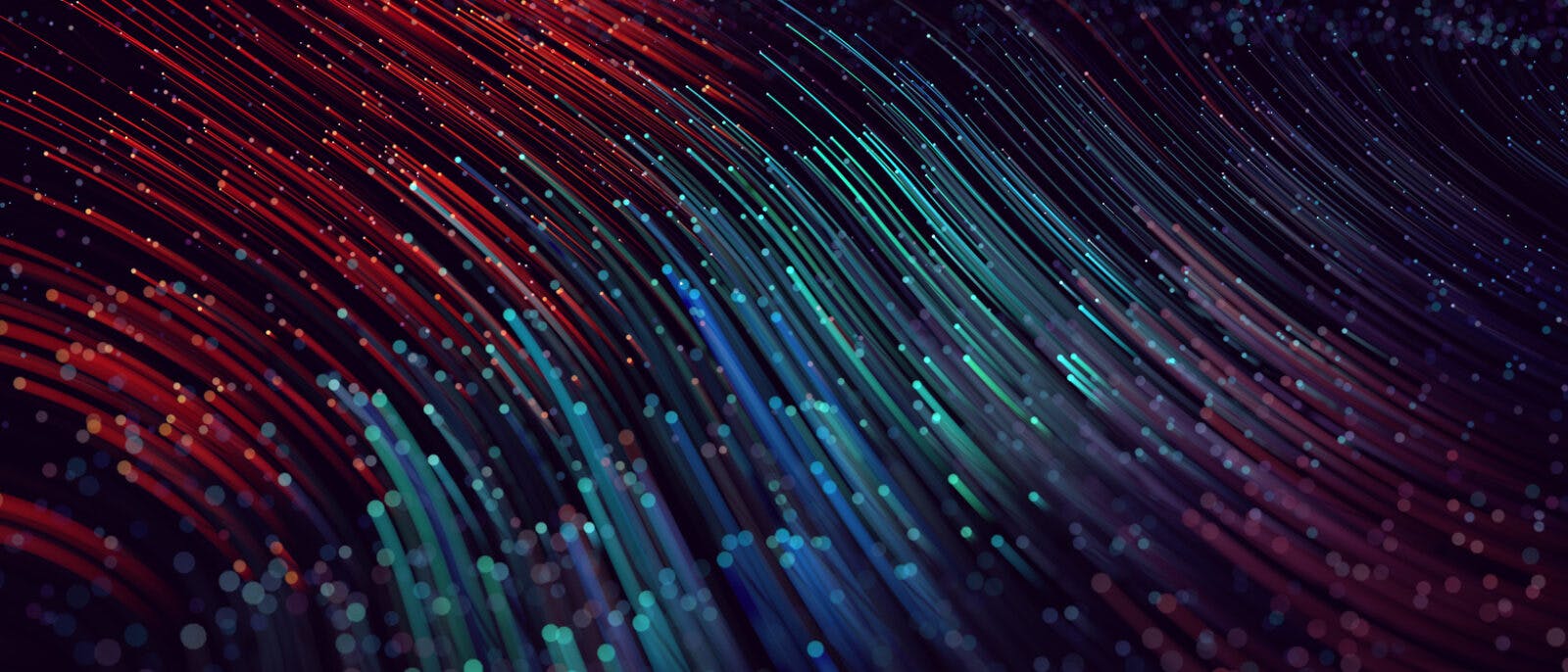
The Author
The Researchers
Yet silicon microprocessors have begun to show signs of age, and for all its flexibility, silicon may be part of the problem. The latest chips, for example, produce so much heat that silicon cannot dissipate it fast enough, so microprocessors cannot run at their full potential speed without melting. In fact, researchers are already investigating new types of integrated circuits that might solve silicon’s problems by communicating information with light or tapping quantum mechanics for computing. Is silicon up for the challenge, or are we entering a new age? And if so, what might that be?
We invited five experts to discuss the future of silicon. They are:
- Gabriel Aeppli – Director of the London Centre for Nanotechnology (a joint program of Imperial and University Colleges) and whose research is focused on the manipulation of quantum states in silicon. Aeppli recently spoke at the 2014 Frontiers in Nano Science & Technology Conference, which was held at the California Institute of Technology’s (Caltech) Kavli Nanoscience Institute.
- Amnon Yariv – Professor of applied physics and electrical engineering at Caltech. He is a pioneer in photonics and quantum electronics, and a 2010 recipient of the National Medal of Science.
- H.-S. Philip Wong – Professor of electrical engineering at Stanford University. His team developed the first nanotube-based computer.
- Thomas Schenkel – Program head at Lawrence Berkeley National Laboratory, where his group is developing technologies to build quantum computers.
- Andrei Faraon – Assistant professor of applied physics and material science at Caltech. He builds nanoscale devices that enable quantum optical communications on semiconductor chips.
THE KAVLI FOUNDATION (TKF): It seems that every age – Stone Age, Iron Age, Machine Age – has to end sometime. Where does the Silicon Age stand? Are we at the beginning or the end?
H.-S. PHILIP WONG: I believe silicon is not at the middle or the end of its life, but having a midlife crisis and now is poised to do something different. Silicon had a great run the last 30 to 40 years, and that exponential performance would be hard to replicate. But the technology and know-how that we learned from silicon, together with new materials and new ways to use its electronic functionalities, will probably contribute silicon’s second career.
GABRIEL AEPPLI: I agree completely. Silicon’s second life is going to come from becoming compatible with other materials that extend its capabilities. We’ve invested so much in silicon in the past 50 years, it would be very difficult to ignore it going forward.
THOMAS SCHENKEL: I agree as well. There has been a lot of talk about using silicon with other materials, and taking advantage of electronic properties other than charge.

ANDREI FARAON: I believe the next big thing will involve using light to connect processor cores and other blocks of devices on semiconductor chips. The main players, like Hewlett-Packard and Intel, are already investing in it.
AMNON YARIV: Yet silicon is not a good light or laser material. It’s probably one of the worst.
AEPPLI: Yes, and so we have to add other materials to it. Semiconductor makers are investing not just only in waveguides that act like circuits to channel light, but also in active materials that amplify and generate light. People are increasingly learning how to place these materials on silicon substrates.
"I believe silicon is not at the middle or the end of its life, but having a midlife crisis and now is poised to do something different." – H.-S. Philip Wong
YARIV: Yes, some of the latest developments involve bonding silicon to light-generating compound semiconductors, which are crystals of two or more materials. The silicon acts like a waveguide to carry the light. Optical communications within chips will alleviate the problems we have sending electrons over very small, nanoscale wires at high speeds, such as electrical interference and crosstalk. This is something Intel wants to commercialize. My own background is in using silicon for communications. Rather than transmit information as bits of either charge or light, we want to control the frequency of light. This will let us increase the amount of data we transmit, but to do it we need to both guide and store light. We are moving in the direction of silicon-compound semiconductor hybrids to do this.
TKF: Researchers are looking at many materials other than silicon. They range from the compound semiconductors that Professor Yariv mentioned to graphene, diamond, and superconducting materials that carry electricity without any resistance or heating. In fact, Professor Wong’s group recently demonstrated the world’s first computer based on carbon nanotubes. Some may have better properties than silicon. Why are you so sure silicon will play a role in the future?

YARIV: I recently read that the amount of investment in silicon technology runs into the hundreds of billions of dollars. We have not made anything like that investment in any other material system. Based on my own experience, any time something could be done in silicon, it was done in silicon. Even materials systems that looked better than silicon on paper lost out to silicon because the economics and technology are already there. It’s a safe bet that the computing and communications world will revolve around silicon for a long time, though increasingly we are likely to use silicon as a middleman, an enabler of other technologies, to achieve performance we cannot achieve with silicon alone.
AEPPLI: In some ways, silicon is like a vacuum. Although I trained as an electrical engineer, I follow what physicists do, and they like to do physics in a vacuum. Why? Because a vacuum is clean, so you can put atoms in it and manipulate them in ways that win Nobel prizes. For engineers, silicon is as close to a vacuum as you could possibly get with real materials. Because it involves a single chemical element, rather than a combination of elements like compound semiconductors, it forms simple cubic crystals that are easy to make with a high degree of perfection and purity. I can buy commercial silicon wafers on the web with less than one part per billion impurities. That’s far cleaner than anything we ever deal with. Silicon is readily available and inexpensive to manufacture. It is going to remain the vacuum of choice for engineers unless the rules of chemistry and economics change.
"What I learned is that whenever someone wanted to develop something, the first question we would be asked is whether it could be done in silicon, because working in silicon was so much easier." – Andrei Faraon
TKF: It is like building a house and finding only a couple of defects?

TKF: Today’s silicon devices are based on electrical current, moving electrons from one place to another. What other fundamental electrical properties could we exploit that we are not using in today?
YARIV: If talking about light and quantum communication, which will eventually move towards silicon like everything else, then we’re talking about properties that involve waves rather than charge. These include qualities like spin, the direction an electron or the nucleus of an atom spins; polarization, which involves how light waves interact with one another; and entangled states, where paired particles interact with one another even though they are separated in space. Instead of building transistors from millions of silicon devices, quantum computing would use individual atoms or waves of light to store and process information.
SCHENKEL: And when it comes to take advantage of some of these properties, we are fortunate to be working with silicon. We have been implanting ions in silicon to control its electrical properties for decades. It turns out that we can prepare silicon so that when we implant ions in it, those ions will maintain their spins for exceptionally long times, as much as 30 minutes at room temperature. Others are trying to use spin in devices based on conventional semiconductor technology so we could use the manufacturing infrastructure we already have. This might take a decade or more, but it promises to be very exciting going forward.

SCHENKEL: In a way, we’ve just scratched the surface of silicon. Until now, we have built chips using lithography, a process that etches very complex patterns of circuits into silicon. It’s like using a stone axe. Today we are using this axe to make features only a few nanometers in size, but it is still an axe and we are now approaching its limits. But as Professor Aeppli said, there is a very rich quantum layer underneath those limits, and we need to find ways to tap this richer universe.
YARIV: We’ve been doing this for a number of years in the communications world by creating Qdots from compound semiconductors. These are essentially artificial atoms. By changing their geometry and implanting them with various materials, we can change their optical properties and control the interaction between them. Here, the axe not only defines the silicon floor where the current runs, but also the properties of artificial atoms bonded on top of our silicon wafer.
"Silicon is readily available and inexpensive to manufacture. It is going to remain the ...choice for engineers unless the rules of chemistry and economics change." – Gabriel Aeppli

TKF: So it sounds like silicon’s role is changing. In the past, we manipulated silicon to make electronics. Now, we’re using it as a superstructure, a backbone, the way we use steel girders in a skyscraper to hold up glass facades and concrete floors. Will this be difficult transition?
AEPPLI: We’ve been depositing materials on silicon since the early days of semiconductors. We’ve used metals to conduct electricity. Now we’re depositing exotic electrical insulators, like hafnium oxide. Because silicon is so well understood, it is quite easy to give it new functionalities and deposit other materials on top of it.
WONG: Yes, and going forward, it will be possible to go beyond these passive materials and apply materials with intelligence in them.
YARIV: I agree. So far, the insulators and metals we’ve used with silicon were all passive materials. We are now beginning to incorporate active materials that can do things like optical manipulation and light generation. These active devices are the beginning of a new world for silicon.
TKF: So silicon would conduct light and other materials would carry it. Is there an optical version of the transistor that could do some of the processing as well?
FARAON: Optical processing was a hot topic in late 1980’s, but researchers concluded that it was not energy efficient. Optical computing would dissipate more energy than electronics. Optics makes better sense for high speed communications, because less light is lost than electrical current.

TKF: We spoke earlier about taking advantage of the quantum properties of silicon. Could silicon play a role in quantum computing?
YARIV: From the outside, I’ve seen the fashion in quantum computing swing from using spin to polarization states and more recently to superconducting circuits, which my friends tell me are now the hottest candidates.
"In a way, we’ve just scratched the surface of silicon. Until now, we have built chips using lithography, a process that etches very complex patterns of circuits into silicon. It’s like using a stone axe." – Thomas Schenkel
SCHENKEL: Superconductors are very exciting. Their features are so large – 10’s of microns in size – and yet they retain their quantum interactions with one another for microseconds at a time. While that is shorter than the spin times we’ve seen in silicon, we can manipulate superconductors very fast, in nanoseconds. Superconductors are ahead of silicon in terms of our ability to build rudimentary quantum devices. But it’s difficult to see how to create billons of Qbits in superconductors. On the other hand, silicon has demonstrated longer spin times and we can already build billions of conventional devices on silicon. Perhaps we will find a way to combine the two materials and build superconducting devices on silicon to take advantage of long spin times in silicon as a quantum memory and use classical silicon electronics for control circuitry.
TKF: Let’s look at another way of storing quantum information. Electrons can only inhabit a limited number of orbits around the nucleus of an atom. Professor Aeppli, your recent work involves storing information by controlling which orbit the electron inhabits. Could you tell us about that?
AEPPLI: As I mentioned before, today’s highly purified silicon is like a vacuum. Our team is trying to do in silicon what the atomic physicists have been doing with free atoms in a vacuum – push around electron spins and orbits in controlled ways. We can do this with millions of atoms at a time, and make features that are large enough to make with conventional silicon processing. One could conceive of building chips that manipulate and read those orbits. So there’s a ghost of a technological future here — not yet real — that is emerging from the science.

SCHENKEL: It is quite an achievement. Electron beam lithography is a very nice stone axe, especially if it lets us create features that are compatible with the orbital states of atoms. It totally invites us to consider how we might structure materials that take advantage of quantum and optical properties in mass produced chips.
TKF: With so much to be discovered about quantum computing, how do we gauge whether silicon or other materials are the way to go? Is there a map?
AEPPLI: I think we’ve turned a corner. Atomic physicists trap atoms in vacuums and manipulate their quantum properties. If we poured money into this, we could make traps in silicon that enable us to do the same thing. There is an engineering path that one could follow to get there. The same thing goes for superconducting Qbits. They are very large, and their potential for miniaturization is limited, but if we wanted to build larger machines, it’s only a matter of money to try. Pure silicon based machines are not as far along. We haven’t agreed on the best way to create interacting Qbits. But if silicon does take off, it has a better future because we know a good deal more about silicon processing and can make much smaller structures. Any of the leading groups, if they were given $1 billion, could come up with plausible engineering route to building a quantum computer.
FARAON: I’m sure many groups would take on the challenge if given a billion dollars. But it’s questionable if they could build one that truly beats the practical computers we have now. While some aspects of the technology are in principle scalable, each Qbit would need to be carefully aligned and tuned. It’s a daunting problem, even if given $1 billion.
"Instead of building transistors from millions of silicon devices, quantum computing would use individual atoms or waves of light to store and process information." – Amnon Yariv
YARIV: Yes, they are willing to spend government money, but not their own money.
AEPPLI: That’s almost a general theorem.

SCHENKEL: Yet quantum information processing is a much richer universe than classic information processing, and we are just beginning to understand the consequences of that. We’ve applied our existing circuit model with its individual bits, logic gates, and communications to quantum computing, and that might not be the most promising way to go. Maybe we want to take advantage of quantum properties to solve problems in totally new ways, such as quantum annealing, which is based on minimizing free energy, or topological computing, based on braiding quasiparticles. These might be less mature than circuit based approaches, but they are very exciting. Perhaps silicon can play a big role in various aspects of these systems, but we are very much at the beginning.
TKF: Let’s finish by asking each of you about the future. Based on your own research where do you expect to see the most exciting breakthroughs over the next five or six years?
AEPPLI: I think we will solve the problem of spin interaction and create two-Qbit gates, which would function like quantum transistors. This would give us the type of switch needed to a meaningful quantum processor.
YARIV: My research concentrates on using silicon as a high-quality storage medium for light. This will enable us to create a new generation of optical oscillators that we can use for extremely high-speed optical communications, so we can deliver more information faster over the Internet and other networks.
FARAON: I’m focused on making on-chip quantum devices where photons interact with spins. I’m hoping these devices will be used for quantum repeaters, similar to amplifiers we now use to make up for signal losses, so we can do high-speed quantum communications over long distances economically.
WONG: I’m focused on transforming nanomaterials into complete electrical systems. Recently, our group built the first complete computer out of carbon nanotubes. Over the next few years, I’m hoping to further develop these types of systems to achieve not only the functionality but the speed and cost-performance ratio of the best silicon computers in the world. My goal is not to displace silicon, but to develop a complementary technology to integrate on top of silicon.
SCHENKEL: I also believe in the intermediate period we will master reliable spin-based Qbit devices and demonstrate the interaction of these devices in silicon.