Optical Switching and a Surprising Twist
by Alan S. Brown
Research highlights from Kavli Nanoscience Institutes
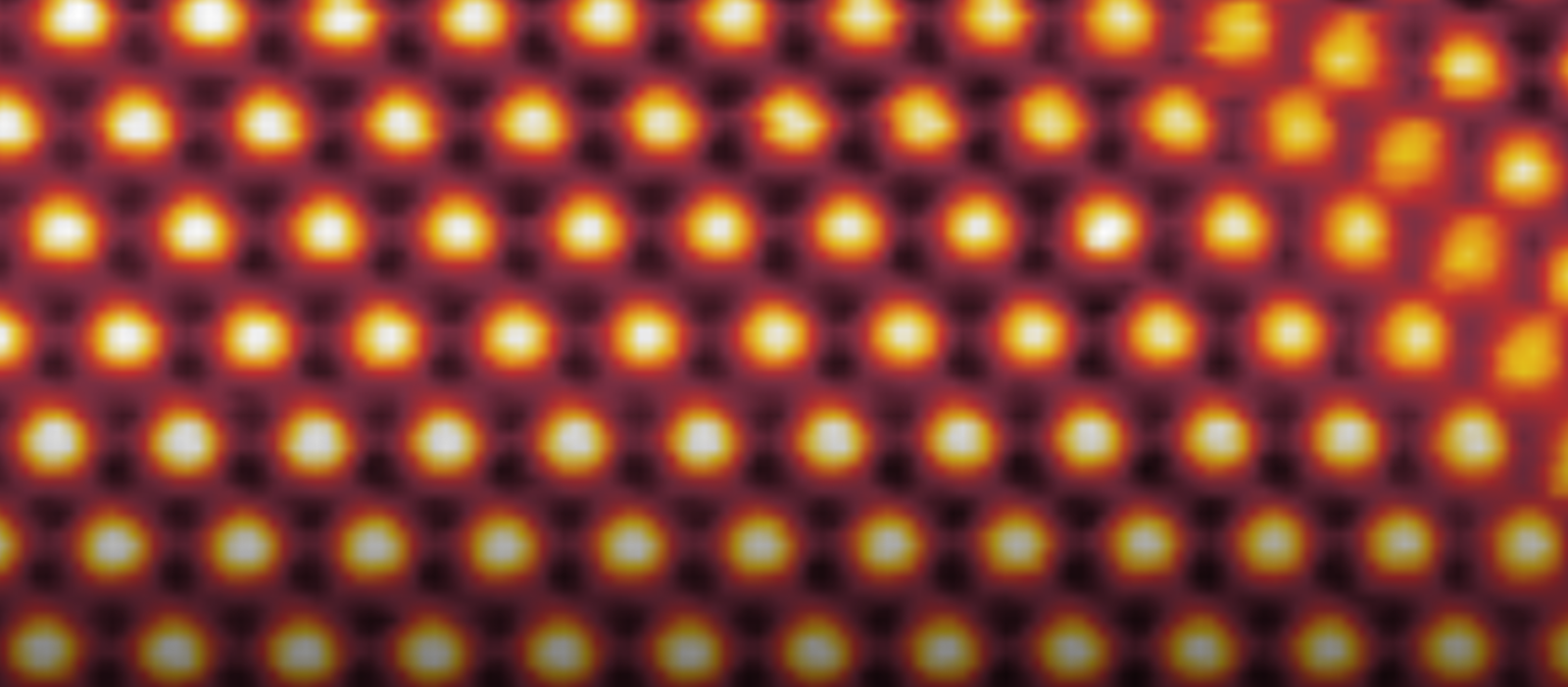
The Author
This month we shine light on a notable advance in optical switching and find some surprising twists in graphene-based moiré superconductors.
Fast, low-energy optical switching
For decades, researchers have been chasing optical computers that would run faster, cooler, and draw less power than conventional electronics. One challenge has been switching, which has been relatively easy in electronic circuits ever since transistors replaced vacuum tubes. Fast photonic switching is far more difficult; until now photonic switches demanded a tradeoff between high power and low speeds. Now, a team led by Alireza Marandi, a member of the Kavli Nanoscience Institute at Caltech, has figured a way around the problem. By utilizing a strong (quadratic) optical non-linearity of thin-film lithium niobate that is not easily obtainable in bulk materials, the team has achieved the fastest low-energy switching yet observed in integrated photonics. The result can enable on-chip, ultrafast energy efficient all-optical information processing. Careful design of the photonic waveguides (passageways for light) allows “dispersion engineering” to create both spatial and temporal confinement of the light. This leads to significant enhancement of the non-linear “photon interaction” effects. allowing this prototype device to achieve switching speeds of 50 femtoseconds at energies as low as 80 femtojoules, about 1,000 times faster than electronic switches and with an order of magnitude lower energy-time product than previous optical switches.
Superconductor shows surprising twist
Moiré materials are made by stacking a few single-atom-thick layers of material with their latices twisted with respect to each other. In the past few years, three layer stacks of graphene assembled with the “magic” twist angle of 1.5 degrees have been found to poses robust and surprising correlated electron states, including superconductivity, that can be tuned using an electric field applied by a back-gate. Kavli Nanoscience Institute at Caltech researcher Stevan Nadj-Perge has discovered that there are at least two types of superconductivity in this material, and at least one of the types has novel, unconventional properties that will require new theory to understand. The team used high-resolution scanning tunnelling spectroscopy in conjunction with a back-gate to control the carrier density in material and reveal transitions and peak-dip-hump structures in the conductivity that is more consistent with bosonic-coupled modes than with traditional “cooper-pair” superconductivity models. These discoveries will enable further understanding of superconductivity and other electron correlations in moiré materials and beyond.
Tabletop pursuit of quantum gravity
At a quantum level, matter and energy—from photons and electrons to electromagnetic forces and even vibrations—are comprised of discrete components called quanta. It’s everywhere we look, but so far, no one has found evidence of quantum gravity. In a fascinating Caltech interview, Kavli Nanoscience Institute member Rana Adhikari and theoretical physicist Kathryn Zurek discuss how they hope to detect ripples in the fabric of spacetime on a desktop. In some ways, their experiment resembles the $1.1 billion Laser Interferometer Gravitational Wave Observatory (LIGO), whose two 2-mile-long arms first identified gravitational waves seven years ago. The desktop version will use the same configuration as LIGO, but its arms will run only a few meters in length. To make it work, Adhikari and Zurek’s team will need to create unusual quantum states and make the most precise measurement of distances ever.
Architecting nanostructures
Julia Greer, director of the Kavli Nanoscience Institute at Caltech, is a worldwide authority on creating complex structures from nanoscale materials. Starting with rods as building blocks, she has built open lattices that resemble the structure of the Eiffel Tower or some Victorian steampunk fantasy. In a fascinating interview, she discusses how creating such architected structures opens the door to new inventions by uncoupling properties linked together conventional materials. Ceramics, for example, are stiff but brittle, but when Greer squeezes her spongelike ceramic architectures, they spring back into shape. Such changeable materials could, for example, lead to drug systems that release precise amounts of medication under certain biochemical conditions or filters that change color or shape when they need replacement. She envisions intelligent materials that could actually judge if a point of failure could be corrected during 3D printing, or a shirt whose textiles would rearrange themselves, so it feels as comfortable in the California summer as the Norwegian winter.
Oxford lifts the veil behind the paper
Oxford University’s Kavli Institute for Nanoscience Discovery recently launched its “Behind the Paper” video interviews, where professors interview INsD graduate students about recent publications in major journals. INsD director Carol Robinson, for example, talked with graduate student, Siyun Chen, about untangling how rhodopsin, the mammalian eye’s primary photoreceptor, collaborates with other proteins to turn light into chemical signals. Among the challenges: finding rhodopsin signals in a membrane packed with many other proteins and following its rapid chemical evolution after light hit it. By understanding the behavior of receptors in their native habitat, Chen hopes to improve our ability to target them with drugs. Meanwhile, Syma Khalid, a computational microbiologist, interviewed Charlie Buchanan, a graduate student of INsD member Andrew Baldwin, about how the sugars that coat our airways might have helped the Covid-19 virus spread from animals to humans. The paper is a great example of how researchers team up to crack difficult multidisciplinary problems. Buchanan spent much of his time coordinating team members and helping to fill in the knowledge gaps that existed between their different disciplines. The result: a new technique to study the interaction between other viruses, biom†kavlifolecules, and drugs.