Overlooked Heavy-Metal Gases Could Reveal Alien Life
by Adam Hadhazy
Part 2 of our Signs of Life series examines how gases overwhelmingly produced by microorganisms that contain toxic metals might prove to be a powerful means of searching for extraterrestrial life
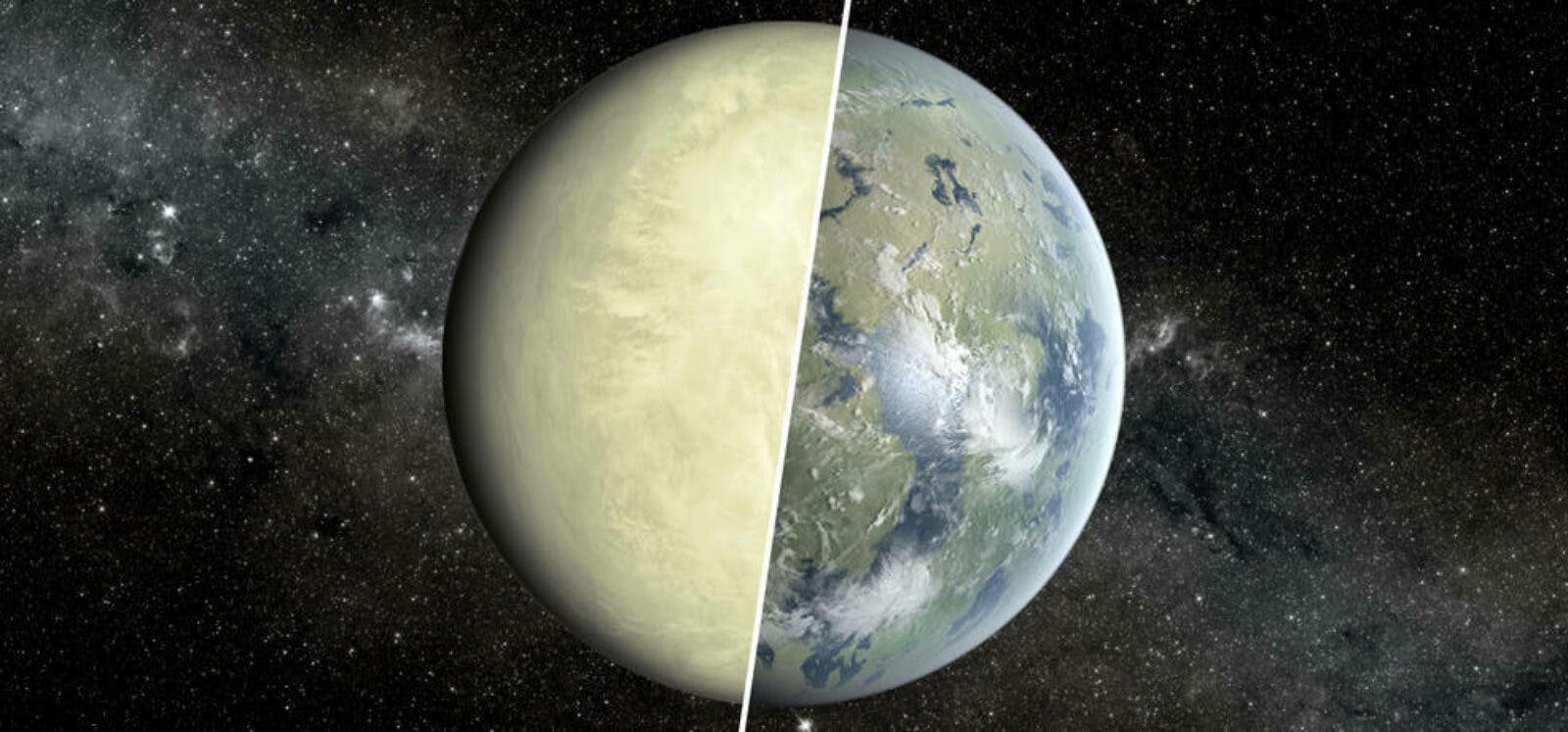
The Author
The search for life beyond Earth is entering an exciting new phase. Over the coming years, researchers will begin observing exoplanets in earnest for "biosignatures"—specific mixtures of atmospheric gases that could be signs of life.
The Kavli Foundation provided support in 2022 for Signatures of Life in the Universe—a Scialog initiative led by the Research Corporation for Science Advancement. At annual meetings, early-career researchers are selected as Scialog Fellows and provided funding for high-risk, high-impact projects by a consortium of funding partners. This article is the second in a series profiling researchers supported by The Kavli Foundation in their efforts to help answer the profound question of whether life exists elsewhere in the universe.
Biochemically, life giveth and taketh. All organisms take in various substances, thereupon giving myriad other substances back into their environments. The reasons for this influx-outflow vary tremendously across kinds of life, from the bare essentials such as eating and excreting and respiration, to more specialized physiological functions like perspiration and pheromone release.
In life's vast chemical milieu, amongst the most unusual emitted products are so-called organometallic gases. This class of substances contains familiar, life-friendly carbon—hence the "organo-" in the name—alongside toxic heavy metals such as mercury, arsenic, and antimony. Only a relatively small number of microorganisms have been documented as producers of these organometallic gases, and for unclear reasons. The gases arise when the microbes interact with the metals and in the process "volatilize" some of them, meaning convert the metals into an easily vaporizable substance. The microbes seem to do this for detoxification purposes, removing the metals from the local environment or from the microbes' single-celled selves.
Counterintuitively, these organometallic gases that are poisonous to life could end up proving as powerful telltale sign of its existence. Giving these neglected organometallic gases their due from a biosignature perspective is the goal of a new project led by Edward Schwieterman and Ziming Yang, the recipients of funds from The Kavli Foundation following the second Scialog: Signatures of Life in the Universe meeting that was held back in June 2022.
A key reason Schwieterman and Yang are pursuing the project is because unlike well-studied biosignature gases such as oxygen and methane, organometallic gases are very rarely made by non-living ("abiotic"), geological processes. In other words, the chances of mistakenly crediting the presence of oxygen and methane in an exoplanet's atmosphere to life are concerningly high. Not so for gases such as dimethyl mercury ((CH3)2Hg) and trimethyl arsine ((CH3)3As). These substances could only reasonably exist in detectable abundance through the machinations of microbial life.
"Organometallic gases are more strongly linked to life than other potential biosignature gases like oxygen and methane, which are also made by abiotic processes," says Schwieterman, who is an Assistant Professor of Astrobiology at the University of California, Riverside. "This new grant received from The Kavli Foundation will allow us to combine laboratory experiments with computer simulations to predict the detectability of organometallic gases on alien worlds with life."
Schwieterman and Yang arrived at the novel idea for their project after meeting and speaking at the second Scialog meeting in 2022. Schwieterman was presenting research on potential gaseous biosignatures, while Yang had been studying the production and transformation pathways of a chemical called methylmercury in environmental contexts such as the Arctic permafrost. "Throughout our conversation," Schwieterman says, "we realized that dimethyl mercury and other organometallic gases hadn't been rigorously examined as potential biosignatures." The researchers further realized that together they possessed the expertise to start looking at the problem.
For the project, Yang—who is an Associate Professor of Environmental Chemistry at Oakland University—is leading the effort to examine the biological production of these gases by organisms in the lab and in their natural environments. Out in nature, organisms cause the emission of these gases by performing a biological activity called methylation. This activity involves adding a methyl group to the inorganic form of the metals and converting them to more toxic and volatile organic metals.
Intriguingly, microbes that expel these metal-loaded gases have been shown to occur across all three domains of life, namely eukarya (who have cells like ours), bacteria, and archaea. That fact suggests the biochemistry required to manipulate these metals is not all that unusual evolutionarily, and if it could work here on Earth, surely it could work elsewhere.
Also intriguingly, why exactly microbes bother to methylate the metals in the first place remains unclear. "It is still largely unknown why microbes are methylating those metals," says Yang. "One thought is that the microbes are doing this process to get rid of toxic metals inside their cells." From previous studies, it is also known that the methylation process could be coupled with other important metabolic processes such as anaerobic (meaning without oxygen) respiration that microbes utilize.
In the lab, Yang is experimenting with organometallic-producing microbes found in Arctic permafrost soils. He is simulating early-Earth and exoplanet environments to figure out how those environments would affect microbes' response and activity to the accumulation and production rate of methylated metals such as methylmercury.
Schwieterman, meanwhile, is leading the atmospheric modeling efforts. Specifically, Schwieterman is determining the minimum detectable abundances of the two gases given conceivable instrumental designs—in other words, how much of the gas would be needed for observers to identify it with astronomical observatories. Furthermore, Schwieterman is gauging the required level of production of these gases to maintain that minimum detection limit.
For the models he is making, Schwieterman is trying out both a modern-day composition of Earth's atmosphere as well as that of an ancient, Archean Earth, dating back to between roughly four and 2.5 billion years ago. Back then, Earth's atmosphere contained very little oxygen. The kinds of microorganisms that nowadays make organometallic gases are all anaerobes, and these organisms are through to have thrived in the Archean era. If so, the microbes could have potentially loaded the atmosphere with the telltale signs of their existence, and such environments could well exist on some exoplanets.
"The anoxic environments that prevailed on Earth billions of years ago, before the rise of oxygen on our planet, may have facilitated a greater microbial production rate of organometallic gases," says Schwieterman.
Besides planets like Earth in its younger eons, the other celestial abodes where Schwieterman and Yang theorize that organometallic biosignatures could abound are worlds circling red dwarf stars. These stars are the coolest, dimmest kind of star in the universe. They are also by far the most common, making up around three-quarters of all stars, and exoplanet discoveries around red dwarfs are increasing apace.
Red dwarfs shine far less brightly than stars like our sun in near ultraviolet (NUV) wavelengths, while producing much more energy at far-UV (FUV) wavelengths. UV, a high energy form of light, breaks apart many kinds of molecules in our planet's atmosphere. The resulting molecular fragments are known as reactive species. These reactive species eagerly seek to react with other molecules. In the process, these reactive species destroy organometallic gases, putting a limit on how much the gases can accumulate in Earth's atmosphere.
Red dwarf-hosted worlds would not follow suit. Though they shine more brightly in the far-UV, these wavelengths tend to be absorbed high up in the atmosphere, limiting their impact on the chemistry below. With far less of the more penetrating NUV light streaking into the lower layers of their atmospheres, the exoplanets should build up substantially greater concentrations of organometallic gases. And these higher concentrations would be easier to detect instrumentally, meaning red dwarfs may be where a novel organometallic gas-driven biosignature hunt could hit paydirt.
While organometallic gases are rare on Earth, we hypothesize their abundance could be much higher on Earth-like planets orbiting red dwarf stars," says Schwieterman. "Red dwarfs present a major opportunity to try out this idea of seeking sign of alien life through organometallic gas production."
The researchers expressed their gratitude for how The Kavli Foundation and other funding streams are helping the work progress. Promisingly, the grant has enabled both Schwieterman and Yang to enlist students in the work.
"Because of Kavli's support, my research group has involved students in the atmospheric modeling of these potential biosignature gases," says Schwieterman.
"The Kavli funding also enables my students to participate in this exciting project, especially for undergraduate students," says Yang. "For undergraduate students, they are new to the astrobiology field but participating this collaborative project could be very helpful for them to start thinking why we care about astrobiology. With the Kavli support, students will learn the experimental techniques in the lab and develop their creative thinking, and more importantly, they will have this great chance to pursue their interests in astrobiology toward their future career."
"Overall, I think the Kavli funding will lead to great things," Schwieterman continues, "to maximize our success of finding life elsewhere we need to build as comprehensive a catalog of potentially biosignatures as possible. I’m excited for what the future holds."