Plenty of Room
by Alan S. Brown
Origami robots smaller than a grain of sand, reconstructing how cells duplicate DNA, quantum computing, nonlinear optics, and even mind control
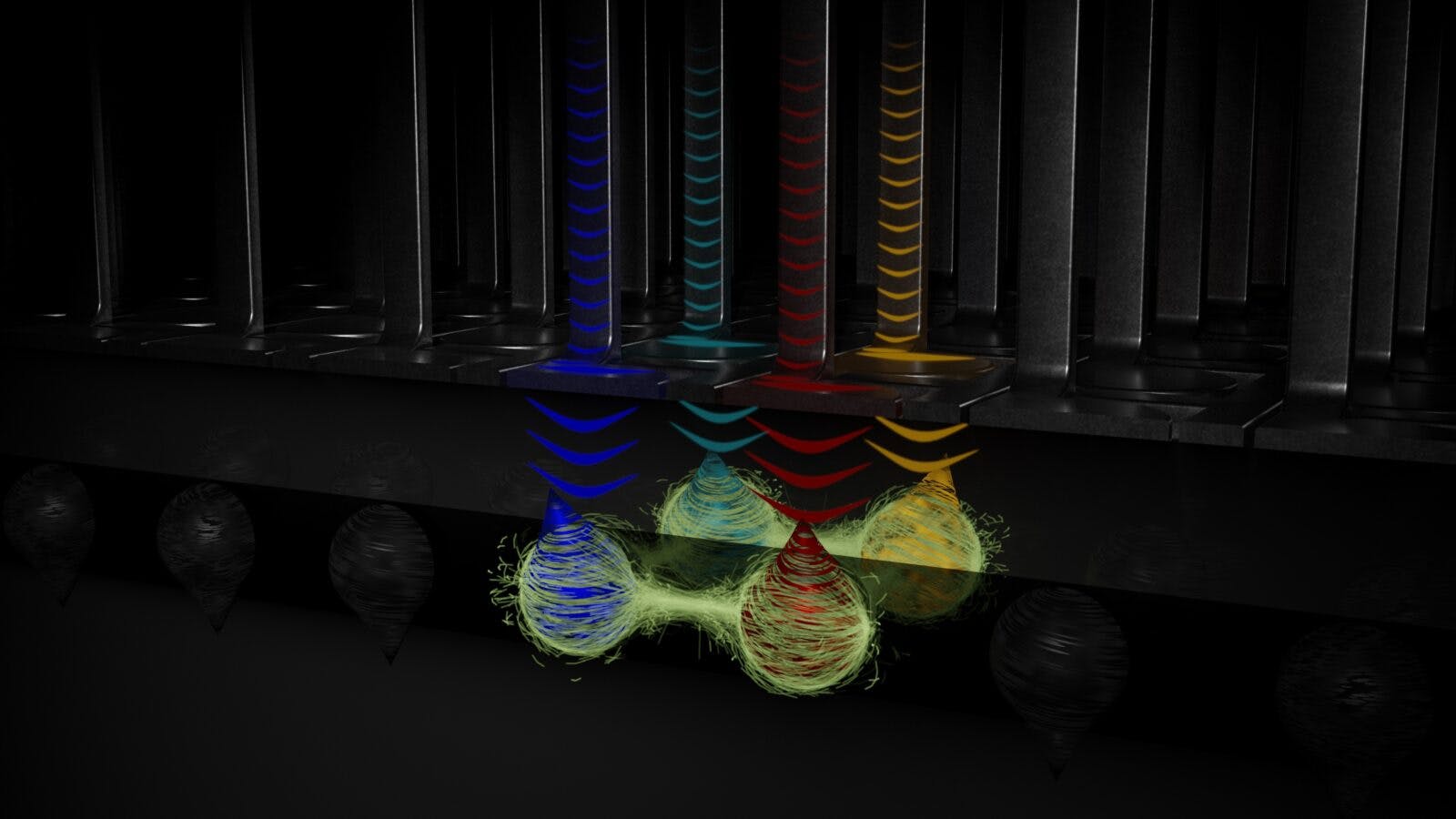
The Author
Many scientists like to trace the start of nanoscience as a field of study back to “There’s Plenty of Room at the Bottom,” Richard Feynman’s address to the American Physical Society in 1959. Feynman envisioned building molecules and devices from the bottom up, much like putting together a Lego castle. He did not think this approach would tell us much about fundamental physics, but it would deliver many new technological applications. While researchers have come a long way in using nanoscience to probe physics and especially quantum physics, this month we focus on applications that would certainly have shocked Feynman. These range from origami robots smaller than a grain of sand and reconstructing how cells duplicate DNA to quantum computing, nonlinear optics, and even mind control.
Origami robot smaller than a grain of sand
Paul McEuen and Itai Cohen of the Kavli Institute at Cornell for Nanoscale Science are known for using origami to create nanobots. Their new creation takes this to the extreme. One-twentieth the size of a grain of sand (60 microns wide), their new origami bird snaps into shape in just 100 milliseconds. All it takes is a single jolt of electricity and the platinum-titanium-titanium dioxide bird will fold itself up and hold that shape indefinitely. Applying a negative voltage returns the 30-atom-thick bird (3,300 times thinner than a sheet of paper) back into its original shape. Next on the agenda, the researchers want to implant semiconductor circuitry, sensors, and a source of power — they have used solar cells in the past — so the robot can perform some basic tasks.
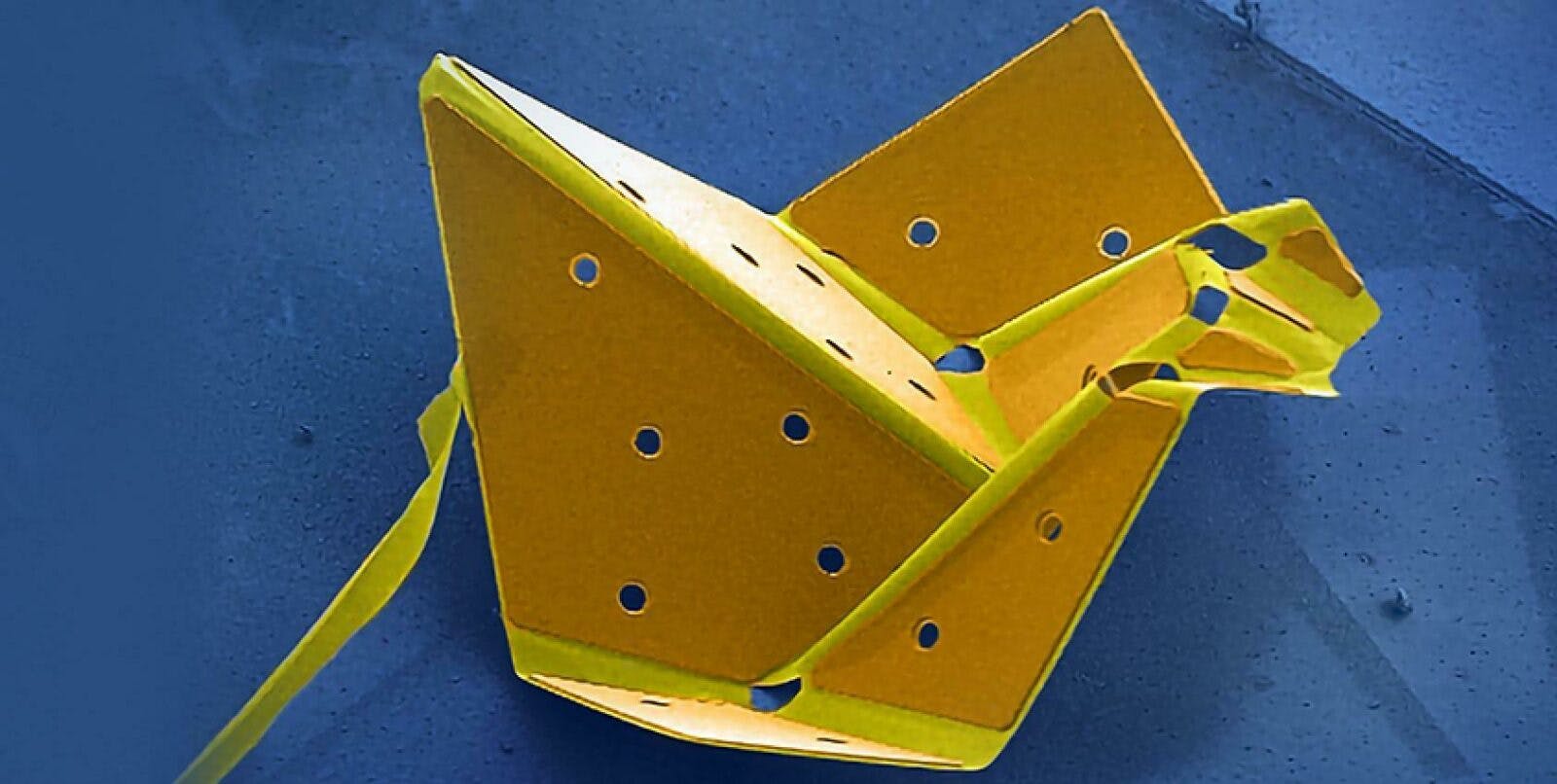
Unpacking DNA
We copy a light-year’s worth of DNA over our lifetimes. Scientists know a lot about the process, but we are far from understanding how the proteins that orchestrate this process place the right molecules in the right place at the right time to do it. Nynke Dekker, a member of Kavli Institute of Nanoscience Delft, and a team of researchers at the Francis Crick Institute, have unscrambled at least one part of the mystery. They started by attaching fluorescent labels to the origin recognition complex (ORC) protein, which begins the construction of the cell’s DNA replication machinery. They then watched as ORC diffused along strands of DNA—and came to a halt when it reached certain DNA sequences to kick off the process. They also discovered that a key “motor” for the replication machinery, know as MCM, exists in a previously unknown mobile form when not attached to ORC. By building this knowledge, Dekker hopes to one day construct a living synthetic cell from the ground up.
Counting to millions of qubits
Researchers have used several technologies to create circuits with up to 53 qubits (the quantum equivalents of transistors). Yet there is no clear way to scale these systems into the equivalent of digital chips packed with hundreds of millions or even billions of transistors. Now, Menno Veldhorst, a member of the Kavli Institute of Nanoscience Delft, thinks he may have found a technique that would scale up to millions of quantum elements. It is based on quantum dots, a technology often associated with high-definition televisions. In quantum computing, these artificial nanocrystals are used to trap an electron that researchers then entangle. So far, they have been unable to entangle more than two qubits. Veldhorst, however, switched to a system that works with holes (missing electrons) in germanium. Using simple circuitry, he has created a four-qubit grid that entangles easily and with very good control. Moreover, germanium is a well characterized semiconductor that interfaces well with other materials (like superconductors) being considered for quantum devices.
A simpler way to control nonlinear devices
Nonlinear devices are systems where one plus one does not equal two. Take, for example, nonlinear optical devices, which change light from one frequency to another. When you add strong nonlinearity to resonators, which trap and circulate light for lasers and other devices, the result is something Kavli Nanoscience Institute at Caltech member Alireza Marandi calls a “rich physics regime." That is another way of saying “really, really complicated,” which is the opposite of what engineers need if they want to design useful nonlinear resonators. Yet Marandi may have a solution for them. While testing a nonlinear resonator made of optical fiber and a nonlinear waveguide, he found that at certain lengths, the light entering the system made abrupt transitions to other frequencies (colors). This finding would enable engineers to tune a nonlinear resonator using just one variable. One day, Marandi speculates, this could lead to optical computers that would count colors the way digital computers count electric charges.
Mind reading
For years, researchers have pursued technologies that would improve the ability of people who are paralyzed to communicate and control their surroundings by controlling a keyboard or robotic arm with their minds alone. The problem has always been reading the mind with enough precision to do something useful. Implanted electrodes in the brain do this, but they are invasive and potentially dangerous. Functional MRI works but requires costly machinery. Electroencephalography (EEG) is neither bulky nor expensive, but it lacks resolution. Now, a collaborative team that includes Kavli Nanoscience Institute at Caltech member Mikhail Shapiro, has developed a way to use ultrasound to read the part of the brain that controls motion planning. It works by measuring the movement of blood flowing through the brain. While still in its infancy, the technology can predict the arm and eye movements of two monkeys with good accuracy. The researchers hope they can develop the technology so that it is one day practical.