The Power of Protein Machinery
At Technical University of Delft, nanoscience is uncovering the secrets of the machines that make our cells run.
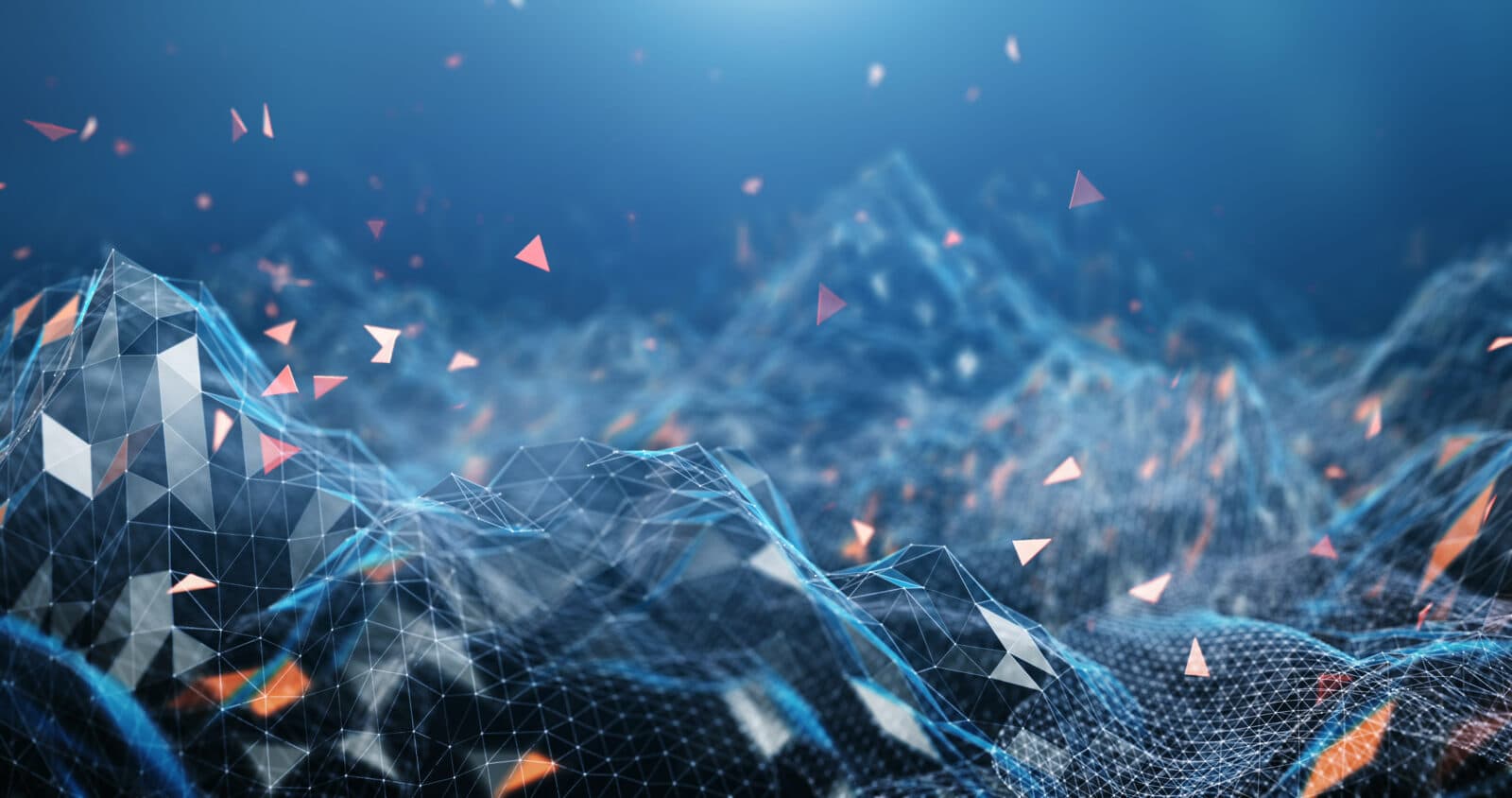
The Researcher
Nanoscience examines some of nature’s most remarkable engineering and nowhere is this engineering more exquisite than in the cell, where thousands of proteins work as tiny motors to power the processes of life. Developed and refined over billions of years of evolution, these tools perform complicated tasks with amazing speed. For example, a cell can create a full copy of itself in just an hour.
Physics meets biology at the Kavli Institute of Nanoscience at Delft University, where researchers study the tool kit of a living cell.

Today, researchers are eager to see if they can find new uses for this ultra-efficient machinery. Cees Dekker leads research in molecular biophysics at the Kavli Institute of Nanoscience at Delft University of Technology -- a science that applies mechanical concepts from physics to the study of the cell’s many moving parts. It's an approach that, in Dekker's words, treats the cell "as a miniature factory that contains a large collection of dedicated protein machines." A professor of biophysics at the Delft University of Technology, Dekker calls this study of the biological tool kit "one of the most spectacular frontiers in nanoscience."
This factory includes enzymes that resemble manmade motors in miniature, with shafts and bearings and rotary motion. There are linear motors that move step-by-step along tracks made from long protein polymers. Some motors are powered chemically by adenosine triphosphate (ATP), the cell’s major energy source. Others use electrical forces. Dekker’s group at the Kavli Institute is studying the many functions of these machines, including their role in DNA repair. It applies nanoscale imaging and manipulation techniques to study how proteins perform what he calls the "complicated DNA gymnastics and long-scale interactions" needed to rejoin broken double strands of the DNA molecule.
A Factory at Work
Cells can mend DNA in two ways, one simpler than the other. The easier fix is to take the ends of the broken molecule and stick them back together, but this is risky because material may have been lost at one of the ends and the reconstituted DNA may be an incomplete version of the original. The other method is less error-prone but much more complex. In it, the broken DNA searches chromosomes to find exactly the right sequence (out of about 3 billion possibilities) and extracts the genetic content it needs. "All of those actions are done by certain motor proteins," Dekker says, from propelling the DNA in its search to invading the chromosome with the needed sequence.

Dekker also studies the coiling and uncoiling of DNA molecules, a key part of gene expression. DNA strands are enormously long in molecular terms – about one meter -- but they normally occupy only one millionth that space, or one micron. They achieve such compression by coiling up like a telephone cord, or, more precisely, being coiled up by protein motors. The motors also uncoil sections of DNA, as needed, so that genetic information can be transmitted to produce proteins or RNA. To get a close look at the dynamics that drive both processes, Dekker and his group use magnetic tweezers to add tiny visible beads to DNA molecules. A microscope can record the coiling or uncoiling motion of the molecules by tracking the beads. Researchers can see the actions of particular proteins on the DNA. Some cause the DNA to uncoil by adding length. Others – motor proteins – will cause coiling.
Motor proteins can also turn against DNA, if the DNA is from a hostile source. Dekker is studying how proteins work to destroy bacteriophages – viruses that invade and feed on bacteria. As in the repair of DNA, motor proteins are involved at all stages of the process. Dekker says they help identify the phages’ DNA then deploy a "restriction enzyme" that "reels in" the phage’s DNA and cuts it. His group uses tweezers and optical beads to see how this defense system works.
Opening New Frontiers
This research suggests a host of potential applications, not all having to do with life as we know it. In medicine, an understanding of the molecular-level mechanics behind processes such as DNA repair might lead to treatments for diseases in which such processes break down or go awry. Several diseases marked by hypersensitivity to sunlight (xeroderma pigmentosum, Cockayne syndrome) and premature aging (Werner’s syndrome) are caused by faulty DNA repair. More broadly, damage to DNA repair genes is associated with high cancer risk. And that covers just one set of cellular tools.
In and beyond the life sciences, protein machinery is being studied for nanofabrication, propulsion of nanoscale devices, or sorting of molecules in "lab-on-a-chip" genetic analysis. Given all that cells can do, including the production of energy from sunlight in a process similar to that of solar cells, there may be near-endless uses for the cells’ workhorse proteins. Biology provides "this great toolbox," says Dekker, that can be used to solve problems that aren’t strictly biological in nature.
If the naturally occurring motor proteins aren’t enough, there’s always the possibility of reverse-engineering machines that do as much or more. In effect, this would be creating at least some components of artificial life, though Dekker says science is far from being able to assemble an entire artificial cell. So as cyborgs are not just around the corner. Dekker believes it’s more likely scientists will learn how to engineer proteins to make bacteria to produce energy (such as hydrogen) and food more efficiently.
Still, while no killer application for bio-nanoscience has yet appeared, Dekker says it will be a huge step forward for science and medicine just to gain "a basic understanding" of the cell’s machinery.