Primordial Portrait of the Universe
Researchers of the Kavli Institute of Cosmology at the University of Cambridge are looking to a new space-based telescope to learn what happened in the first moments of the Universe.
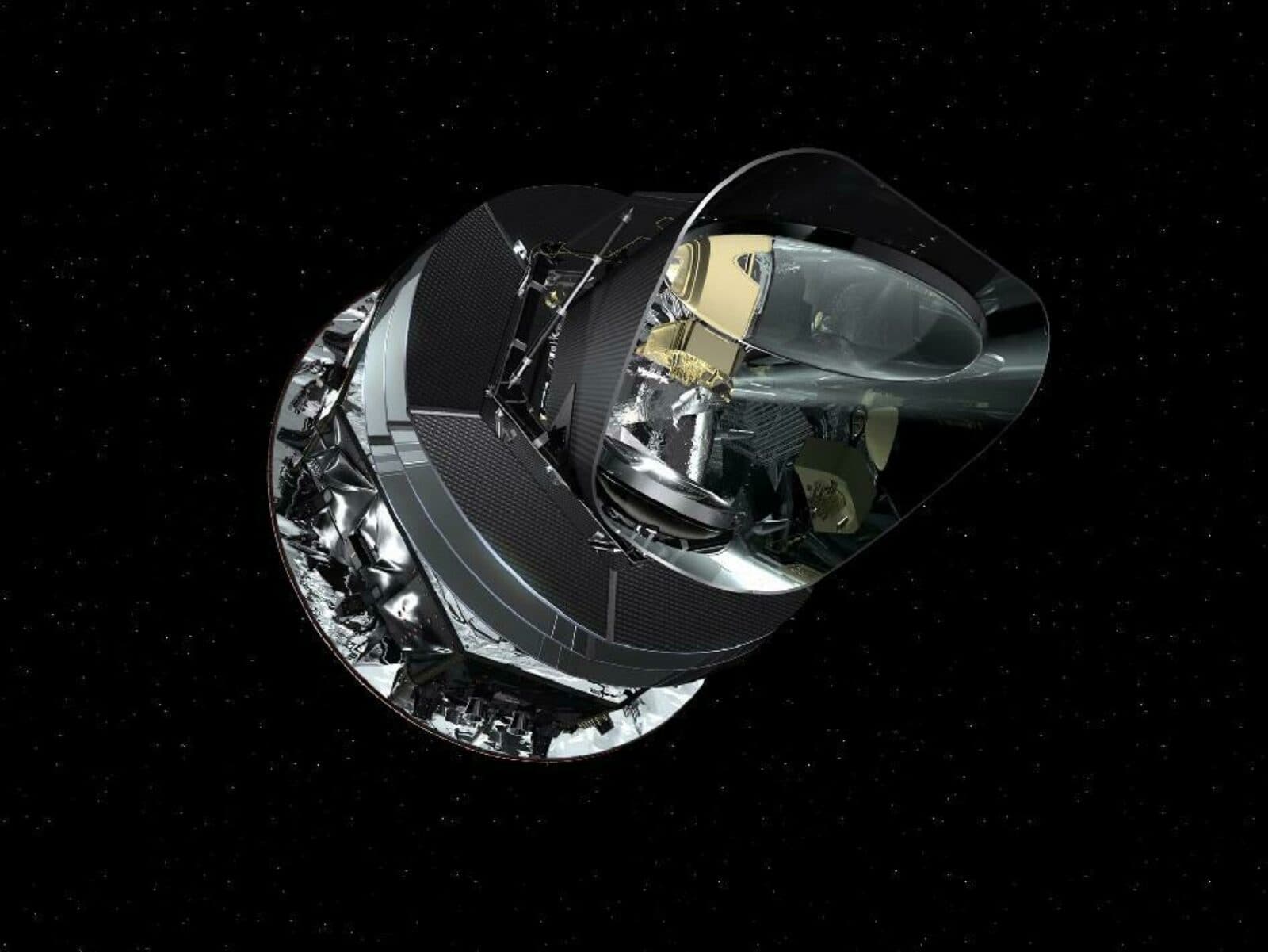
The Researchers
Central to the science of cosmology is the zeal to build better time machines. These are not designed literally to travel to the distant past, of course, but to get a better look at it. The latest of these is the Planck Surveyor satellite, due for launch in 2008. A few months after it starts its journey, if all goes well, Planck will be at an orbital sweet spot about a million miles from earth, transmitting images of unprecedented sharpness showing the radiation left over from the Big Bang.

Artist’s view of Planck heading toward its destination, the second Lagrangian point in space, known as L2. About 2.5 hours after being launched with ESA’s Herschel spacecraft on an Ariane-5 from the Guiana Space Centre, Kourou, French Guiana, Planck separates from Herschel and starts its cruise to L2, where it will arrive in less than 6 months. Image: ESA
Researchers of the Kavli Institute of Cosmology at the University of Cambridge (KICC) played a central role in developing the scientific case for the European Space Agency’s Planck mission. They will again be key participants in the work of analyzing the mission’s data. KICC Director George Efstathiou, a Cambridge professor of Astrophysics, says Planck is one of the Institute’s first “flagship projects” focused on its core mission of studying the early Universe. “Provided that it works, it will produce really exquisite images of the cosmic background radiation in temperature and polarization,” Efstathiou says. “It will define the cosmic background radiation sky.”
The Birth of a Universe
The potential knowledge to be gained from Planck goes far beyond mere images, as good as these may be. Scientists hope to find evidence that sheds light on the very early Universe, as it was around 10-35 to 10-33 seconds after its birth. Most cosmologists believe that something happened in this unimaginably brief period to make the universe expand with enormous speed – much faster than the speed of light – rather than collapse into itself under the force of gravity, like a black hole. This “inflation,” as it’s called, would have swelled the universe from a diameter vastly smaller than a proton to that of a grapefruit. That’s still puny, compared to its size some 13.7 billion years later, but the force expanding it to that point would have been enough to break the hold of gravity and allow it to continue expanding from that point.
Why is inflation important? And what can Planck tell us about it? One answer to the first question is that, the more we know about how the Universe started, the more we understand about how it works now, at the largest and smallest of scales, and what its future may look like. In particular, studying the inflationary epoch may help resolve the differences between the physics of the very large, such as Einstein’s theory of General Relativity, and quantum mechanics, where sub-atomic particles follow laws that seem quite different from those that govern the stars and galaxies. Planck’s role in unlocking the secrets of inflation will be to record small irregularities (called anisotropies) in the temperature and polarization of the cosmic microwave background (CMB), which covers the whole sky at a temperature less than three degrees above absolute zero.
A "Quantum Leap" in Resolution
When the CMB was discovered in 1965, most scientists took it as convincing evidence that the Big Bang had indeed taken place. Since then, they have been studying it for clues about the just what happened in those first moments of the Universe. Although the CMB was not generated right at the Big Bang – it dates from about 300,000 to 400,000 years later – it carries the signature of that primordial event. The forces that first gave the Universe its structure are believed to be reflected in the CMB’s anisotropies. Those fluctuations were first discovered by Cosmic Background Explorer (COBE), and satellite launched by NASA in 1989, and they were mapped in more detail by another NASA mission, the Wilkinson Microwave Anisotropy Probe (WAMP) launched in 2001. Earth-based observatories also have contributed to our knowledge of the CMB, including the Degree Angular Scale Interferometer (DASI) in Antarctica and the Cosmic Background Imager (CBI) in Chile.

Artist’s view showing the Planck satellite and the microwave radiation being collected by the telescope’s primary and secondary mirrors. The mirrors focus the radiation at the focal planes of the two instruments (LFI Low Frequency Instrument and the HFI (High Frequency Instrument). LFI is designed to convert the lower-energy microwaves into electrical voltages. HFI works by converting the higher energy microwaves to heat, which is then measured by a tiny electrical thermometer. Image: ESA – AOES Medialab
Efstathiou says space-based instruments have a great advantage of working in a “clean environment” with little interference, and WAMP has been “incredibly successful” at imaging the CMB with radio frequency detectors. But he expects Planck to achieve a quantum leap in resolution by using bolometers, super-cooled sensors that precisely measure the heat of radiation.
In producing its high-resolution map of the CMB, the Planck observatory will deliver the most precise measurement yet of anisotropies. Scientists then can analyze these fluctuations to see how they match up with the patterns predicted by various models of inflationary physics. They will be looking at the largest-scale fluctuations, associated with the earliest phase of inflation, for signs of a rapid expansion followed by a sharp slowing. Planck may also be able to spot another sign of inflation, a gravitational wave (on a scale with the whole Universe in length) that shows up in the polarization pattern of the CMB.
The Planck mission is expected to run about five years, with up to three years for data collection and two for data analysis. Efstathiou says a “tight-knit” team of KICC scientists representing all three Kavli-affiliated departments in Cambridge has been working on the Planck project. These include Professor Anthony Lasenby and Dr Mike Hobson of the Cavendish Laboratory, Professor Neil Turok and Dr Paul Shellard from the Department of Applied Mathematics and Theoretical Physics, and Efstathiou himself.
Efstathiou is the U.K. principal investigator for the mission and is also on scientific teams overseeing the satellite’s high-frequency and low-frequency instruments.
Planck is just one of several research initiatives related to KICC’s mission of observing and understanding the early Universe. One in the works is a ground-based effort to detect the gravitational wave from inflation (it will operate in Chile’s Atacama Desert). The Institute also is involved -- as a contributor to the design of instruments or facilities – to projects focused on the period during which the first stars and galaxies formed. And to ensure the fullest and most creative collaboration, KICC scientists will soon have a new building, due to be built in 2008, designed to bring them together for their KICC work. Efstathiou says this is a first for Cambridge, where cosmology researchers traditionally been housed separately in a physics building (Cavendish) and the Institute for Astronomy. Between the two will sit the new KICC building, providing the space for what Efstathiou calls a “critical mass of cosmologists.”