Reshaping Graphene’s Future
by Alan S. Brown
Graphene can morph from conductor to semiconductor and back again just by changing its geometry. This could open the door for high-performance computing and nanoscale quantum devices.
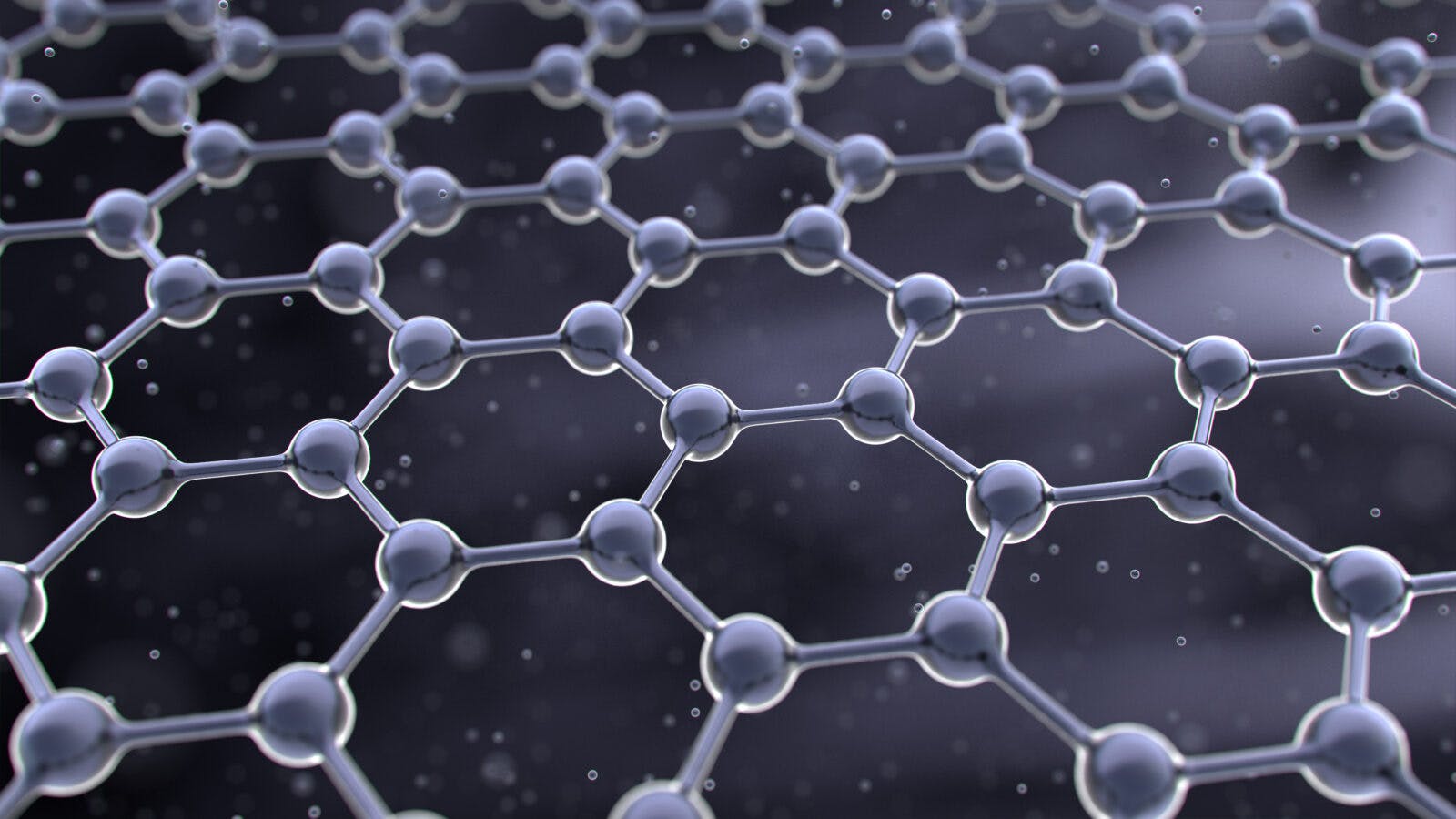
The Author
The Researcher
Silicon electronics dominate the world, but engineers frequently turn to other types of semiconductors to do things that silicon cannot. These materials—ranging from silicon carbide to indium gallium arsenide—emit and interact with light, enable high-frequency microwave communications in smartphones, run at higher speeds, and handle massive amounts of power.
Yet, what if a single material could do all that—if it could interact with electrons, light, and even magnetism. What if it could transmit electricity like a metal and also behave as a semiconductor. Just for good measure, suppose it might make a good building block for quantum computers? And—most impressively—what if it could do all these things just by changing its shape?
That material is graphene, an atomically thin form of carbon, said Michael Crommie, co-director of the Kavli Energy NanoScience Institute at UC Berkeley, and a leading expert on graphene’s electronic properties.
Crommie’s latest research involves transforming thin strips of graphene, called nanoribbons, into electronic devices. Ordinarily, graphene conducts electricity 70 percent better than copper. Nanoribbons, on the other hand, are always semiconductors—until now. By finding a way to transform nanoribbons back into conductors, Crommie now has the elements needed to build an all-carbon transistor and perhaps one day an all-carbon computer.
Bandgaps
Graphene is an outlier in the materials world because it is not a bulk material, one whose thickness can be as deep as its width or breadth. Instead, it exists as a skein of carbon only a single atom thick, often referred to as a two-dimensional material. Unlike other materials, whose properties are dominated by their bulk, graphene’s properties are based on surface interactions.
This gives rise to very different behaviors than silicon, Crommie said. When silicon atoms form crystals, their electrons align in triangular pyramids. When those electrons bond with other silicon atoms, they produce a rigid, three-dimensional crystal with fixed electronic properties.
Graphene, on the other hand, has three electrons that lie in a plane, each 120 degrees from the others, while a fourth electron sits perpendicular to the plane. When those planar electrons bind to other carbon atoms, they form two-dimensional arrangements of hexagonal rings. Those flat hexagonal sheets give rise to vastly different electrical behavior than found in three-dimensional silicon.
One of the most important of these electronic properties is bandgap, which is what determines if a material is a semiconductor. It is the amount of energy an electron needs to break free of the atom and conduct electricity. Metals have no bandgap and so their electrons move through them easily. Silicon is a “semi” conductor because it only conducts when its electrons have enough energy to break way from their atoms and begin to move.
Graphene conducts electricity like a metal, but at the nanoscale it has some unusual properties. Electrons moving within graphene’s two-dimensional networks of bonded carbon rings form waves. When researchers worked out the details, they realized that if they could make graphene small enough, it would interact at a quantum level with these waves and alter its electronic properties. One of those properties was its bandgap.
“If you trap electrons in a narrow sliver of graphene called a nanoribbon, the bandgap gets larger,” Crommie said. “Make the nanoribbon wider and the bandgap gets smaller. Change the zigzag or armchair pattern formed by the carbon hexagons on the edge of the film and the electronic properties change.”
In other words, make a graphene nanoribbon of the right size and shape and it would behave like a semiconductor. All researchers had to do was build it.
Nanoribbons
Building nanoribbons is not a simple task. Researchers could not simply slice a sheet of graphene into ribbons with a laser. No matter how precise the cut, it would create imperfections, especially along the edges, that would destroy the fragile material’s electronic properties.
Instead, they had to build their nanoribbons from the ground up by encouraging chemicals to self-assemble into the right shape. “I went to my chemistry friends and asked them whether I could get a molecule that would produce a zigzag edge and most of them had no idea,” Crommie said.
His search eventually led him to fellow Kavli Energy NanoScience Institute member and chemist Felix Fischer. After painstaking research, Fischer eventually developed with a molecule that would produce a graphene nanoribbon in two steps. First, it would polymerize to form a single layer of carbon-carbon bonds. When heated, it would then give up the hydrogen atoms on the nanoribbon’s edges. The result was a perfect zigzag (adjacent closed carbon rings) or armchair (alternating open and closed carbon rings) edge.
“It took a lot of chemistry to get both reactions to happen efficiently and perfectly every time,” Crommie said. “Eventually, we learned how to get surprisingly long, beautiful, defect-free nanoribbon segments, a level of control that you could never achieve without self-assembly.”
Theory stated that all nanoribbons were semiconductors. But to make transistors and other electronic elements, Crommie needed to transform at least some of these nanoribbons back into conductors. This would give him the two essential elements needed to make a transistor, the basis of all digital computing.
Once again, Crommie started from the bottom up. He realized that semiconducting nanoribbons acted like insulators to prevent electrons from moving. But if he could place nanoribbon segments with different geometries next to one another, their differing electronic properties could trap an electron at the interface between them.
“We can build up a metal from those electrons,” he said. “If we get a sequence of electrons that are perfectly evenly spaced, they can jump from space to space and that’s exactly what a metal is.”
Fischer built a molecule that produced that geometry and the researchers demonstrated the first conducting graphene nanoribbon in September 2020.
Now they had all the elements needed to make a transistor and other electronic devices. Crommie believes it might one day be possible to make an entire integrated computer with properties far superior to silicon from circuits made from a single sheet of graphene.
The ability to control electronic properties by altering graphene’s shapes could also open the door to quantum computing. Crommie is working on ways to control magnetism within nanoribbons. This might enable him to create qubits—the quantum analog of transistors—in very small structures and devices.
In a world dominated by silicon electronics, he believes, graphene offers new and often surprising alternatives.