The Science of Sugar Cravings
by Lindsay Borthwick
Despite the COVID-19 pandemic, brain research marches on
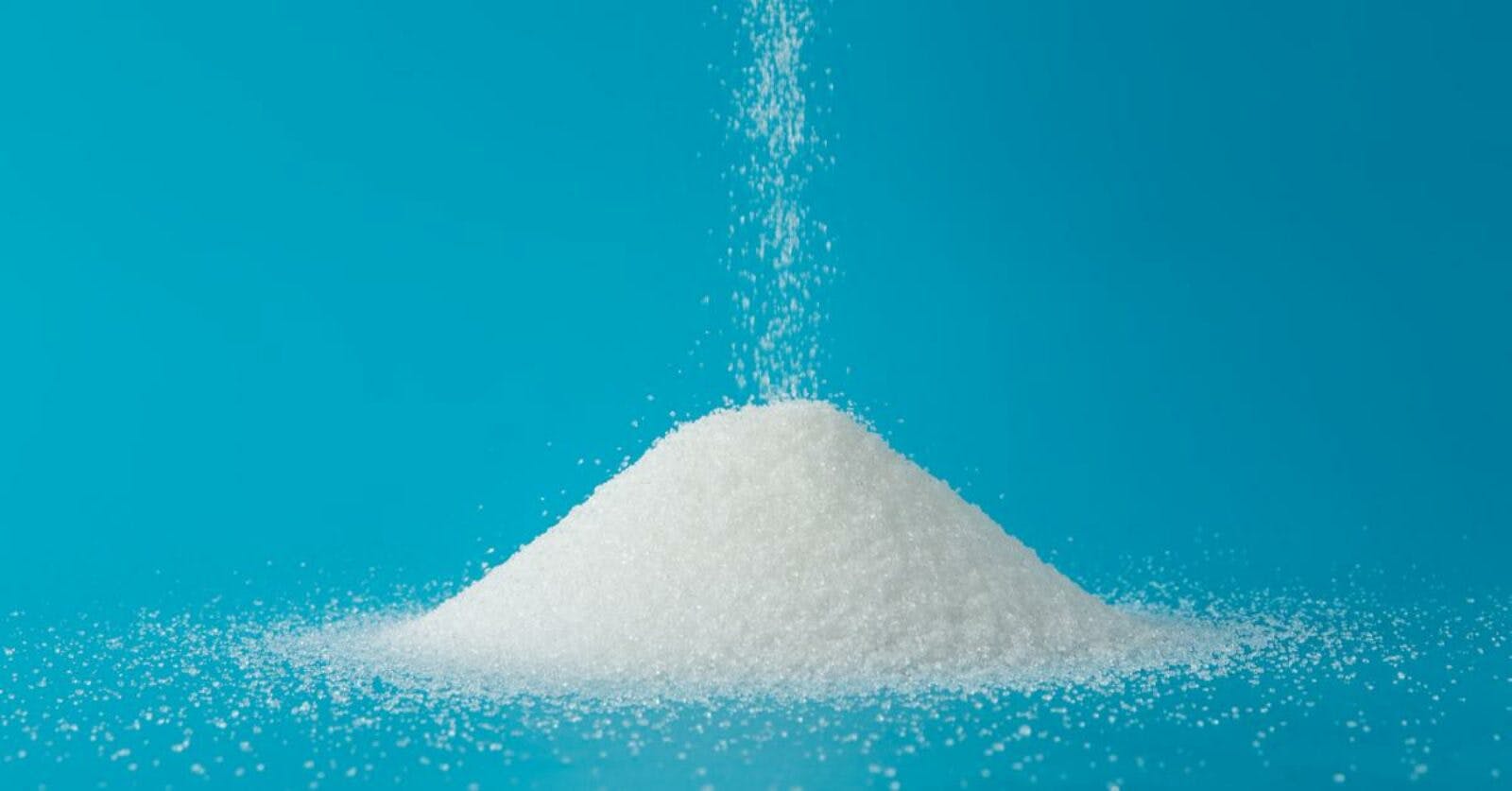
The Author
The Researchers
Despite the COVID-19 pandemic, brain research marches on. We have heard from the Kavli community that they are still busy doing the stuff of science—analyzing data, writing up results, reviewing grants and research papers—and also taking advantage of the change in pace to reflect and plan new research projects. Some are also pivoting their work to contribute to tackle the COVID-19 pandemic. The following research highlights will give you a sense of their rapid progress toward understanding the nervous system—and how some neuroscientists are applying their skills in surprising new ways.
Those constant cravings, explained
Sugar has a secret. Yes, it delights the tongue, triggering sweet-taste receptors that send signals to the brain. But it also stimulates nerve cells in the gut, according to new research led by Charles Zuker, a neuroscientist at Columbia University and a member of the Kavli Institute for Brain Science. A series of experiments in mice shows that sugar molecules, not artificial sweeteners, trigger a neural pathway connecting the intestine to the brain, where they drive the urge for even more sugar. This observation suggests there is a neural basis for sugar preference that is distinct from sweetness. In fact, when the team fed sugar water and artificially sweetened water to mice, the animals switched to drinking only sugar water within two days. By closely examining their brain activity, the researchers made a surprising discovery: A population of cells that responds only to the presence of sugar in the gut. The cells are located in an area of the brain stem called the caudal nucleus of the solitary tract. Incoming signals travel from the lining of the intestine to the brain via the vagus nerve. "Uncovering this circuit helps explain how sugar directly impacts our brain to drive consumption,” Zuker said in a statement. “It also exposes new potential targets and opportunities for strategies to help curtail our insatiable appetite for sugar.”

Adult neurons have what it takes
Dr. Mark Tuszynski, a clinician and neuroscientist at the University of California, San Diego, has published results that he called “downright shocking” in a statement. Tuszynski, who is an expert in spinal cord injury and repair, discovered that injured adult brain cells revert to an embryonic state. Furthermore, he found that from this immature state, the cells are capable of regrowing axons, the slender projects that transmit electrical signals, when provided with a permissive environment. Reporting this month in the journal Nature, Tuszynski and his research team describe what they call the “regenerative transcriptome,” the array of genes expressed by adult corticospinal neurons after injury and during regrowth. Though the research was conducted in mice, it suggests that the adult nervous system in mammals is dramatically more resilient than previously thought. Tuszynski is director of the Translational Neuroscience Institute at UC San Diego School of Medicine and a member of the Kavli Institute for Brain and Mind.
Making sense of smell
A scent is not a simple thing. Even a familiar, everyday aroma like a morning coffee contains hundreds of individual odors. A longstanding question for neuroscientists is just how the brain can distinguish so many different of them. A new study, co-led by Stuart Firestein and Elizabeth Hillman at Columbia University and enabled by a powerful 3D brain imaging technology, overturns a major assumption about olfaction. It showed that the brain doesn’t simply interpret a complex odor as the sum of its parts. Instead, odor receptors, which are located in nerve cells in the olfactory epithelium, deep within the nose, shape the sensory signals the brain ultimately receives, influencing how smells are perceived. For example, one odor in a mixture can change how a nearby cell responds to a second odor, either enhancing or suppressing the cell’s response.
Until now, researchers didn’t think this type of odor processing was happening so early in the olfactory system. But in this study, they could actually see how thousands of different cells lining the nose responded to different odors. Using a high-speed microscope called SCAPE, developed by Hillman, they were able to simultaneously monitor the activity of 10,000 cells in live mice. “SCAPE provides a major advantage in that, because we can watch such a large area at one time, we can catch important events that happen rarely,” Firestein told the NIH, which partly funded the development of SCAPE through the U.S. BRAIN Initiative. This technique has opened the door for us to explore many additional questions in the olfactory field.” (Hillman is a member of the Kavli Institute for Brain Science at Columbia, which contributed funding to the study.)
Calming the cytokine storm
Joshua Vogelstein, a neuroscientist and biomedical engineer at Johns Hopkins University, usually studies animal and machine intelligences. In mid March, he began applying his machine-learning and data analysis skills to a new problem: COVID-19. Vogelstein’s father, a Hopkins’ professor who studies cancer genetics, had previously discovered that a common class of drugs could prevent hyperinflammation, also known as the “cytokine storm,” in mice. He thought the drug may prove useful for treating some patients with COVID-19 who progressed to pneumonia and acute respiratory distress. So Joshua pulled together a team of researchers to examine whether the drug prozasin, a common and well-tolerated alpha-1 adrenergic receptor antagonist, had helped patients survive cytokine storms in the past, by analyzing thousands of hospital records. Their preliminary results were promising, so the team is launching a clinical trial to test whether prozasin can improve outcomes for people with a severe form of COVID-19. Vogelstein is a member of the Kavli Institute for Fundamental Neuroscience.
An ACE in the hole
Rockefeller University neuroscientist Erich Jarvis, a member of the Kavli Neural Systems Institute, is striving to understand how spoken language and other complex behaviors evolved, how they are learned and the brain changes that permit vocal learning in some species. To do so, he studies a variety of animals, including songbirds, looking for molecular and genetic differences between them. He is now applying that lens to COVID-19. Specifically, he is studying ACE2, the receptor used by the novel coronavirus to infect host cells. Many animals express the receptor, but not all of them are susceptible to infection. Jarvis wants to know why. He is searching for structural differences between the ACE2 receptor across different species that could explain their susceptibility to infection.