Sculpting Optical Microstructures With Slight Changes in Chemistry
(Originally published by Harvard SEAS)
March 30, 2017

In 2013, materials scientists at the Harvard John A. Paulson School of Engineering and Applied Sciences (SEAS), the Wyss Institute of Biologically Inspired Engineering and Kavli Institute for Bianano Science and Technology (KIBST) grew a garden of self-assembled crystal microstructures. Now, applied mathematicians at the SEAS, Wyss and KIBST have developed a framework to better understand and control the fabrication of these microstructures.
Together, the researchers used that framework to grow sophisticated optical microcomponents.
The research is published in Science.
When it comes to the fabrication of multifunctional materials, nature has humans beat by miles. Marine mollusks can embed photonic structures into their curved shells without compromising shell strength; deep sea sponges evolved fiber optic cables to direct light to symbiotically living organisms; and brittlestars cover their skeletons with lenses to focus light into the body to “see” at night. During growth, these sophisticated optical structures tune tiny, well-defined curves and hollow shapes to better guide and trap light.
Manufacturing complex bio-inspired shapes in the lab is often time consuming and costly. The breakthrough in 2013 was led by materials scientists Joanna Aizenberg, the Amy Smith Berylson Professor of Materials Science and Chemistry and Chemical Biology, a Core Member of Wyss and former postdoctoral fellow Wim L. Noorduin. The research allowed researchers to fabricate delicate, flower-like structures on a substrate by simply manipulating chemical gradients in a beaker of fluid. These structures, composed of carbonate and glass, form a bouquet of thin walls.
What that research lacked then was a quantitative understanding of the mechanisms involved that would enable even more precise control over these structures.
Enter the theorists.

Inspired by the theory to explain solidification and crystallization patterns, L. Mahadevan, the Lola England de Valpine Professor of Applied Mathematics, Physics, and Organismic and Evolutionary Biology, and postdoctoral fellow C. Nadir Kaplan, developed a new geometrical framework to explain how previous precipitation patterns grew and even predicted new structures.
Mahadevan is also core member of the Wyss Institute and a member of KIBST.
In experiments, the shape of the structures can be controlled by changing the pH of the solution in which the shapes are fabricated.
“At high pH, these structures grow in a flat manner and you get flat shapes, like side of a vase,” said Kaplan, co-first author of the paper. “At low pH, the structure starts to curve and you get helical structures.”
When Kaplan solved the resulting equations as a function of pH, with a mathematical parameter standing in for the chemical change, he found that he could recreate all the shapes developed by Noorduin and Aizenberg — and come up with new ones.
“Once we understood the growth and form of these structures and we could quantify them; our goal was to use the theory to come up with a strategy to build optical structures from the bottom up,” said Kaplan.
Kaplan and Noorduin worked together to grow resonators, waveguides and beam splitters.
“When we had the theoretical framework, we were able to show the same process experimentally,” said Noorduin, co-first author. “Not only were we able to grow these microstructures, but we could also demonstrate their ability to conduct light.
Noorduin is now a group lead at the Dutch materials research organization AMOLF.
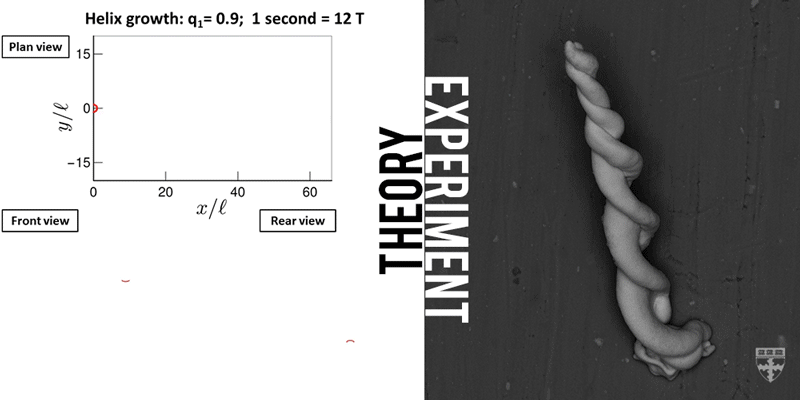
“The approach may provide a scalable, inexpensive and accurate strategy to fabricate complex three-dimensional microstructures, which cannot be made by top-down manufacturing and tailor them for magnetic, electronic, or optical applications,” said Joanna Aizenberg, co-author of the paper and Director of KIBST.
“Our theory reveals that, in addition to growth, carbonate-silica structures can also undergo bending along the edge of their thin walls,” said Mahadevan, the senior author of the paper. “This additional degree of freedom is typically lacking in conventional crystals, such as a growing snowflake. This points to a new kind of growth mechanism in mineralization, and because the theory is independent of absolute scale, it may be adapted to other geometrically constrained growth phenomena in physical and biological systems.”
Next, the researchers hope to model how groups of these structures compete against each other for chemicals, like trees in a forest competing for sunlight.
The research was coauthored by Ling Li, Roel Sadza and Laura Folkertsma. The research was supported by the National Science Foundation, the Kavli Institute for Bionano Science and Technology at Harvard University and Harvard MRSEC.