Searching For The First Stars
How did the first stars and galaxies bring the young universe out of its dark ages and into the light? Three prominent researchers discuss how new instruments and observational techniques may find the answer.
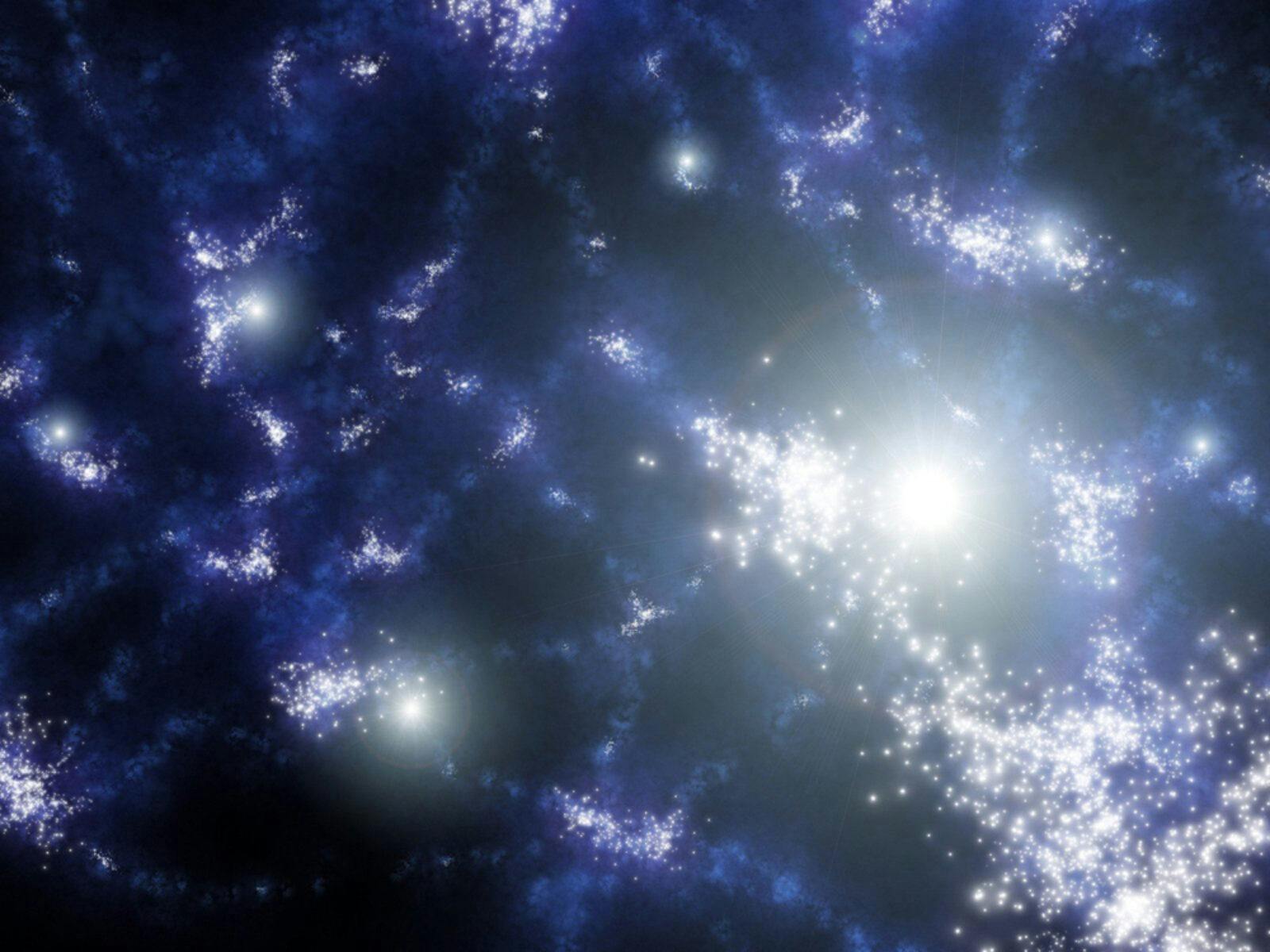
The Researchers
IN NOVEMBER, ASTRONOMERS ANNOUNCED they had found two immense clouds of pristine gas, nearly 12 billion light years away. Formed two billion years after the Big Bang, the clouds appear to be made of hydrogen and little else – no heavier elements such as carbon, oxygen, iron and silicon that later generations of stars cast into space as they exploded in supernovae. These two clouds of gas, astronomers suspect, are the stuff from which the first stars were born.
The discovery was one of several last year in the quest to lift the curtain on the universe’s “dark ages,” an epoch that theorists say dominated for several hundred million years after the Big Bang when the universe was shrouded in a fog of neutral hydrogen gas. These dark ages are believed to have ended only after radiation from the first stars and galaxies “burned” off this fog – stripping neutral atoms of hydrogen of their electrons and ionizing the gas. As the fog cleared in the great voids between galaxies, light from the first stars began to propagate through the growing universe. The epoch of hydrogen reionization – which actually returned hydrogen atoms to a state that existed soon after the Big Bang – gives a scientific explanation to the biblical passage “Let there be light.”
But how did those first stars form, what did they look like, and how did they assemble into galaxies? How did they ionize the vast sea of hydrogen gas that filled the universe? And how did the first generation of stars give rise to the elements that formed dust, asteroids, comets, planets, water – us?
The Kavli Foundation spoke with three prominent researchers about the hunt for the first stars and galaxies, and how direct and indirect observations are helping them get closer. The participants:
- George Becker, a fellow at the Kavli Institute for Cosmology at Cambridge University in the U.K. (KICC), studies the large-scale evolution of matter in the early universe. He specifically studies reionization in the early universe – in part by studying quasars, the energetic cores of galaxies – and how the universe became enriched with heavy elements.
- Richard Ellis, Steele Professor of Astronomy at the California Institute of Technology in Pasadena, CA, studies the emergence of the first stars and galaxies through a variety of observational techniques. Ellis has employed the Hubble Space Telescope, Keck Observatory in Hawaii, and the gravitational lensing properties of foreground galaxy clusters to study distant objects.
- Avi Loeb, Chair of the Department of Astronomy and Director of the Institute for Theory and Computation at Harvard University, studies a broad range of topics, including the first stars, the epoch of reionization, and the formation and evolution of massive black holes.
The following is an edited transcript of a teleconference, which was conducted at the end of 2011.
THE KAVLI FOUNDATION (TKF): Dr. Loeb, you’ve said that the quest to tell the full story of the universe is like assembling a photo album, and that cosmologists are working to fill in the missing pages. These include the epoch when the first stars and galaxies formed. Why is this part of the album so important?
AVI LOEB: These are very important pages. We can see galaxies like the Milky Way forming all the way from one billion years after the Big Bang until the present time. But we don't see the beginning of the story. It's similar to seeing adults on the street, without ever knowing what they looked like as babies and infants. Obviously an adult is not a scaled up version of an infant. Along these same lines, we would like to understand how the galaxies that we see around us, including the Milky Way, came into existence. It's going back in time to our origins, in a sense. Religious texts try to address these questions, but now we are able to come up with a scientific version of the story of Genesis. We would like to collect the data that will allow us to say how the very first stars and galaxies formed, and eventually how they became galaxies like the Milky Way that have stars like the sun. So, it's tracing our origins to very early times, to only hundreds of millions of years after the Big Bang.
TKF: These missing pages in our photo album include the dark ages that preceded the formation of the first stars and galaxies, and the epoch of reionization when their light began to propagate freely. Why have these early times been so difficult to study?
GEORGE BECKER: We’re talking about a point in time that is so far back that the light coming to us from objects that existed then is now very, very faint. But in addition to that, earlier than about one billion years after the Big Bang the universe is opaque to ultraviolet light. And that’s the very light that we commonly use to study these first galaxies, as well as the diffuse gas in which they reside.
Nevertheless, we learn about this early time in several ways. I’ll describe two “archaeological” approaches. But first, you have to imagine the first galaxies living in a sea of gas that acts like a very thick fog. The period dominated by this hydrogen fog is known as the dark ages. But when these first galaxies form, they burn off the fog. The gas in the universe becomes ionized – atoms in the gas are stripped of their electrons – and that makes the gas transparent to ultraviolet radiation. And this is how the universe is for all of time after reionization. Earlier than one billion years after the Big Bang, however, we need to use other tools to study the gas and galaxies.
To study this early epoch, one approach I’ve taken is to look at some of the lasting effects that these early galaxies have on their surroundings, on this gas between galaxies in what’s called the intergalactic medium (IGM). I observe some of the brightest objects in the universe, which are called quasars. They are bright enough that the gas along the line of sight leaves distinct shadows that can be interpreted like a fingerprint or a barcode. And this gives you information about the state of the gas along the line of sight. We know from these observations that after about one billion years all of this gas is ionized, and it's the galaxies that we think did this. The question that we are trying to answer is how and when it happened, and what these earliest galaxies were like.
In addition to ionizing the gas, the galaxies heat it. This is a lasting effect that can be studied even after all the action has taken place. It's like walking up to a stove, and if the stove is still warm then you know it was on recently. And if it is cold, then you know it's been switched off for some time. So we examine the gas in place more recently than one billion years after the Big Bang, and we try to determine whether it's still warm or not. There are some early indications that the stove is still a bit warm, and so maybe all of this action happened not much earlier than one billion years after the Big Bang.
My team has made similar measurements spanning later epochs, when quasars appear in large numbers and reionize all the helium in between galaxies. The reionization of helium atoms also heats the gas, and we've been able to very clearly detect this. It's like global warming on cosmic scales. The effort now is to push those measurements even further back into the past, to study hydrogen reionization.
TKF: So, you use one approach for studying the reionization of hydrogen gas in the early universe, and another one to study the reionization of helium that occurred afterward?
BECKER: The stars that live in galaxies in the early universe emit photons with sufficient energy to reionize hydrogen. To reionize helium, however, you need higher energy photons to break electrons away from helium nuclei. And those photons come from quasars. As quasars become more numerous in the early universe, you should see this helium reionization occurring. And indeed that's what we seem to have uncovered by looking at the temperature history of the universe. We see the universe warming up with time, and then cooling down once the reionization of helium has finished.
TKF: Is the heating of the IGM, then, a kind of proxy for the presence of the first stars and galaxies?
BECKER: That's right. Studying the heating of the IGM is useful for two reasons. Number one, we can measure it relatively well. Secondly, this heating has a lasting effect. For reasons that I'm sure Richard and Avi will describe, it’s difficult to study those first billion years directly. So we also examine the state of the universe afterward – in other words, this heating of the IGM – and we make inferences based on archaeological evidence.
TKF: Richard, you’ve pursued other strategies for indirectly studying the first stars and galaxies, including using the gravitational effect of a cluster of galaxies as a lens that magnifies even more distant galaxies behind the cluster. How is new technology and new observational techniques now pushing your studies in new directions?
RICHARD ELLIS: What we’re trying to do here, to follow Avi and George, is to try to understand whether there are sufficient numbers of galaxies in the early universe to reionize the neutral hydrogen gas that filled the early universe. We think that reionization was probably an extended process that took several hundred million years to complete. For reionization to have happened, the hydrogen atoms in deep space had to be ionized, and those atoms had to be prevented from recombining back into neutral hydrogen atoms. If the energy from young galaxies is sufficient, then hydrogen atoms will be split up into their constituents – protons and electrons. But, left to themselves, a proton and electron in a dense intergalactic medium will combine back into a neutral hydrogen atom – if there is not a sustained output of photons, or light particles, from young galaxies.
By observing foreground clusters of galaxies, we were able to gain our first glimpses of galaxies far behind them. That’s because a cluster galaxies acts like a giant gravitational lens that magnifies even more distant objects seen through it – as you mentioned. These early galaxies had redshifts of 7 and above – which dates them to the first 800 to 900 million years of cosmic history. Our studies combined the power of ground-based telescopes, the Hubble space telescope, and the magnifying property of foreground clusters of galaxies. Our group tried this strategy for many years, and we found a number of convincing candidates for the earliest galaxies.
However, the subject has moved on dramatically during the last two years. A new infrared camera onboard the Hubble space telescope, called the Wide Field Camera 3, has done a number of surveys of deep areas of sky. We now have collectively, through community efforts, about 100 candidate galaxies beyond a redshift of 7. That compares to only a handful that were found much earlier through the gravitational lensing studies. You can say the lensing studies paved the way, but they didn't really deliver the kinds of statistics that we now have through the wonderful camera on Hubble.
As we gather more data about these early objects, we're interested in knowing several things. First, were there more galaxies at early times than at later times, or were there fewer? You would expect that as you get back to the beginning, when galaxies are forming for the first time, their number per unit volume – that is, the number of galaxies – declines. And broadly, that's what we’re seeing, and that's been confirmed with the new camera on Hubble.
"We would like to understand how the galaxies that we see around us, including the Milky Way, came into existence. It's going back in time to our origins, in a sense. Religious texts try to address these questions, but now we are able to come up with a scientific version of the story of Genesis." – Avi Loeb
But the intriguing result that I think first came out of the lensing work, which is now clearly confirmed from the new Hubble data, is that there are many more small, feeble galaxies than large luminous galaxies at early times. If you were to take a space ship and go back to that point in cosmic time, you'd find that the sky is really ablaze with them – so many of them that their combined output is very significant. These early objects are in fact very, very small – about a tenth the diameter of the Milky Way. And yet, they are forming stars more prodigiously than the galaxy we live in today. We know that these early galaxies couldn't sustain that level of star formation for very long, so we are indeed seeing them at an important time in cosmic history. These very low luminosity or “dwarf galaxies,” producing stars at a very high rate, appear to be a very important population.
Now, we can calculate how many galaxies of a certain luminosity you would need to ionize the hydrogen gas in the early universe. But not all galaxies are going to have the same luminosity – some will be feeble, as I’ve described, and some will be luminous. So, we need to know the luminosity distribution of galaxies during reionization. We have other questions we hope to answer: How exactly do the photons get out of these galaxies? How do they interact with the hydrogen atoms? Can they do this in a way that maintains the reionization and heats up the intergalactic medium in a way that George described?
In addition to examining the more direct Hubble images we expect to have in 2012, there are other indirect ways to help us answer these questions. If we fast-forward in cosmic time, maybe 200 million years after the universe was reionized, we can use the Spitzer Space Telescope to do a census of how many stars existed in galaxies at that time. Now all the stars that were in place at this later time must in some sense represent an inventory of what happened during the earlier reionization epoch, which is much more difficult to probe.
Another indirect probe of star formation during reionization is to study the rate of gamma ray bursts – the spectacularly energetic explosions that occur at the end of a massive star’s life. About 6 or 7 of them have been found that date back to these very early times, and even with a handful one can make some deductions about the rate of star formation at that time. There's plenty of hope that within the next few years, we'll find many more gamma ray bursts during this unexplored era.
So the astronomer today has many tricks up his sleeve. It's not just a question of using Hubble. It's a question of working with theorists, using the Spitzer telescope, and studying gamma ray bursts. It’s a very exciting time observationally, with the real promise of filling in the missing link as Avi mentioned. Of course, it’s tough work pushing back the frontiers. I think if you look at the history of astronomy and of telescopes all the way back to Galileo – there have been nearly 400 years of pushing back the frontiers, driving to larger and larger telescopes to satisfy man's curiosity. In some ways, this is the final frontier – the connection between the Big Bang and the very first objects, and how those first objects link to the galaxies that we see around us today.
"The astronomer today has many tricks up his sleeve. It's not just a question of using Hubble. It's a question of working with theorists, using the Spitzer telescope, and studying gamma ray bursts. It’s a very exciting time observationally." – Richard Ellis
TKF: We have talked about searching for the first galaxies, or at least learning indirectly what they were like, but what about the first stars? Computer simulations a decade ago suggested that the first stars were hundreds of times more massive than the sun, and that they formed in isolation from other stars. But more recent studies suggest they were much smaller and arose in groups.
LOEB: So let me begin by offering a few words of background, about the theoretical predictions for how the first stars formed. We have a clear view of the initial conditions of the universe. The cosmic microwave background, which was the relic radiation left behind from the Big Bang and dates to 400,000 years after that event, is like the Rosetta Stone of cosmology.
We can read off temperature fluctuations in the microwave background, which tells us about the initial conditions that the universe started from, and we can then fit in those initial conditions into a computer simulation. But we need to assume something about the nature of most of the matter in the universe – and that assumption is that it’s dark matter, which does not seem to interact with light or with ordinary matter. So computer simulations that predict how the first stars formed must include the presence of dark matter.
It turns out that because ordinary matter started from hydrogen and helium, and there were no heavier elements in the Big Bang, the gas could not cool very efficiently. The result of this is you end up with relatively big clumps of ordinary matter that made the first stars. This is what the computer simulations from a decade ago showed, which supports the theory that the first stars were very massive.
More recent simulations, however, have found that the gas does fragment – it makes a disk and the disk fragments into clumps that could have different masses, going all the way down to smaller masses. The problem, however, is that these simulations are not powerful enough to follow the evolution of this disk for a sufficiently long time. At the moment, then, these numerical simulations cannot tell us the final answer. They can only go midway and say, “We see clumps, some of them are small, and some of them are big. But we don't know what the end result would be.” I would say that we don't have the final word yet, and that there was probably a spectrum of masses of stars that formed early on.
The most massive stars are interesting because they can explode and we can see those explosions individually in the form of gamma ray bursts, which are the brightest explosions in the universe. In those explosions, the core of the star collapses to make a black hole, and in the process of making the black hole jets are being produced. These jets penetrate through the envelope of the star and emit radiation that we can see – all the way out to the edge of the universe. We also expect more conventional explosions called supernova. For very massive early stars, there is a special type of supernova that may have resulted called a pair-instability supernova. We haven't seen one yet at high redshifts, but we could in principle see one. So that's one way to tell if indeed there was an overabundance of massive stars – by seeing them, one at a time, explode.
However, one would like also to see the effects of those early stars in galaxies on the rest of the universe – as we’ve discussed. The breakup of hydrogen atoms into their constituent electrons and protons – that is, reionization – is the biggest effect we’ve discussed. Reionization of the universe happens in a way that is not uniform. In other words, when galaxies form they first break the hydrogen around them, and then these ionization fronts propagate outward and you get the rest of the volume being ionized. One way to find out how this process progressed is actually to image the hydrogen, which emits radiation with a wavelength of 21 centimeters. We can use radio telescopes to detect this emission from hydrogen at cosmological distances.
Eventually, we'll be able to image hydrogen in the early universe in three dimensions – across the entire sky and also back in time along our line of sight. What we expect to see is a bubble structure, similar to Swiss cheese, where you have holes in the “cheese.” The cheese is the hydrogen, and the holes are being produced by the galaxies that break up the hydrogen in their vicinity. The size of the holes is expected to grow with cosmic time, to the point at which these holes overlap and you have no cheese left.
By imaging the hydrogen, we should be able to tell how galaxies influenced their environment in the early universe. This isn’t just about putting our hands to the stove and feeling (or measuring) the heat left behind, which is an indirect method of probing how reionization happened. Instead, it’s actually monitoring how the stove is burning in the early stages. It's a more direct way of seeing what really happened.
There are several groups right now building observatories that would be sensitive to this radio emission by hydrogen, and it's a race right now. These groups are competing with each other, and whoever detects the signal first will get the glory.
"One of the grails would be to directly observe a population of metal-free stars – that is, examine them by the light that they emit. It turns out these earliest generations of stars are expected to produce a different mixture of elements by the fusion in their cores, compared with later generations of stars such as those we know and love today." – George Becker
TKF: George, would your work, mapping the thermal history of the IGM, be able to detect spatial variations in temperature at specific points in cosmic time? That would be evidence for this “Swiss cheese” structure, right?
BECKER: Under special circumstances, yes. And these would be to look at the gas immediately around our background targets – which are quasars. Around a quasar, you have a very large hole in the cheese, and you get just a glimpse of the gas and a little bit of information about its temperature. But quasars are incredibly rare in the early universe. And so, this kind of method would only work in a very loose sense. If you go a little bit further along, as the universe ages, you’ll have more of these quasars and more regions of the universe to look at. You can then check if there are variations in the later temperatures. It's sort of like having a series of stoves, and checking the temperature of each one.
But what Avi is describing is really quite a bit more fundamental toward directly determining whether the hydrogen has been broken up or not. It is more direct than measuring variations in temperature in the IGM, and also significantly more difficult. So, what Avi is describing are exciting measurements that will be made over the next several years.
TKF: Avi, why is this direct measurement of 21-centimeter radiation so difficult?
LOEB: Let me start with George's statement about why this is exciting. Most of the volume in the universe is actually at large distances. Our goal has been to map the distribution of matter at the largest distances, which happens to be at the early times that we’re talking about. To date, existing surveys have imaged only a tenth of a percent of the observable universe, so this effort to image hydrogen gas at the largest distances is truly a frontier. It will offer us a major improvement of our understanding of the epoch of reionization, and allow us to map the biggest chunk of the universe that we have ever mapped.
In terms of the technical difficulty, the problem is that the radio signal from hydrogen is relatively weak, and there are emissions from other sources closer to us. If you put these observatories on Earth, TV and radio stations in cities emit the same type of radiation at the same frequencies – which, by the way, correspond to the FM band on your radio. So you want to put the experiment away from such interference. But even when you go up into space, you’ll find that our galaxy emits radiation very brightly at these low frequencies; it emits a background that is 100,000 times brighter than the signal that we are after. So we need to clean up this contamination.
Fortunately, these foreground radio emissions from the galaxy are smooth as a function of frequency. By contrast, the signals that we are after have very sharp fluctuations as a function of frequency. This is because each frequency that we are observing corresponds to a different distance in the universe. In our effort to map hydrogen gas in the IGM through its 21-centimeter line at ever increasing distances, we are slicing the universe at different redshifts, and each redshift has a corresponding observed frequency. As a result, we expect the signal to actually fluctuate a lot as you move from one frequency to a neighboring frequency. The radio noise from the Milky Way will not show this fluctuation, so even though this noise is extremely bright we should be able to remove it.
TKF: One major question is when and how stars began producing elements heavier than hydrogen and helium – the elements that make up dust, rock, planets, and eventually life. When and how do you expect we’ll detect this important generation of stars, and learn about how the elements that make us arose?
ELLIS: We would dearly like to know the chemical composition of the stars in the galaxies we see at very early times, but unfortunately this is beyond the limit of our current capabilities. What would be a clincher, in terms of determining whether these were “first generation” objects, is if we could see stars with no evidence of heavy elements. Elements like carbon and oxygen were not produced in the Big Bang, which only produced hydrogen, helium and a few other light elements. The heavier elements that we see around us – including the ones that make us up as individuals and what we see around us on Earth – are all synthesized in the nuclei of stars. And so if one goes back far enough in time, a measurement of the first generation of stars would show a pristine star cluster with no evidence of heavy elements.
TKF: And we haven’t detected this yet?
ELLIS: No. This would require spectroscopy at very high redshifts, and at these high redshifts all of the energy is shifted into the infrared. We really need powerful facilities operating at infrared wavelengths. The James Webb Space Telescope (scheduled to be launched by about 2018 as a successor to Hubble) will have a spectroscopic capability that will take us out to when the universe was only 400 or 500 million years old. Next generation ground-based telescopes like the Thirty-Meter Telescope, the Giant Magellan Telescope, and the European Extremely Large Telescope (all anticipated to see first light by 2020) will take us out to a period of about 600 million years after the Big Bang.
Theorists have some doubt as to whether these pristine stellar populations are detectable. Why? Well, let's try to think how this happens. A star is born. It's made only of hydrogen and helium. It has no carbon or iron in its atmosphere. So therefore, it sends a very characteristic signal that we might see with the James Webb Space Telescope. But the earliest populations of stars may be very massive, and so they may finish their lives very quickly. A massive star can complete its cycle in only a few tens of millions of years, and then produce a supernova. And when a supernova explodes, all the nuclear fusion that has occurred in that star during its brief life will very quickly pollute the gas that is in the star cluster, so that subsequent second-generation stars that form in that star cluster – which is happening all the time because these early star clusters are forming stars at a prodigious rate – will of course already contain the products of the supernova explosion.
And so it may well be that this is going to be a very difficult measurement, to clinch this quest for the very first generation of stars and galaxies. But it's certainly one for which we'll need observational data, and that's why these future facilities are going to be so important.
BECKER: Certainly one of the grails would be to directly observe a population of metal-free stars – that is, examine them by the light that they emit. It turns out these earliest generations of stars are expected to produce a different mixture of elements by the fusion in their cores, compared with later generations of stars such as those we know and love today. As an alternative to looking for the first stars directly, therefore, we can search for the unique chemical signatures that these stars left behind.
TKF: So what kind of mix of chemical products did these first-generation stars leave behind, according to theory?
BECKER: Well, theory is not entirely settled on what they produced. Even predicting what an ordinary star will produce is quite challenging. However, the consensus is that the mixture from the first stars will be very different. One suggestion is that very massive stars might produce much more silicon relative to other elements, such as oxygen and carbon – when compared with later generations of stars. Again, observing quasars helps us. Quasars not only have shadows cast on them by the hydrogen and helium along the line of sight but by these trace elements that are produced by stars. So we can go back to these very early times, roughly one billion years after the Big Bang, and identify pockets that have been enriched with some of these earliest chemical products of stars. By measuring their chemical compositions, we can make some inference about the stars that produced the heavier elements.
"Early on, when I was younger, I was interested mostly in philosophy, in the humanities – and one of the fundamental questions for humans is how everything started. ...[W]hat attracts me to this topic [is] that I can now go full circle and come back to a fundamental question I considered in my youth – addressing it with theoretical tools and actually finding the answer." – Avi Loeb
At least by one billion years after the Big Bang, it appears that all of the pockets of elements that we see are very similar to the kinds that we would expect to be produced by ordinary stars at later periods in the universe. That is, we don't see these unusual abundance patterns that would've been left behind by the first stars. And this sort of furthers the line that Richard was suggesting – that the earliest generations of stars, these potentially massive but certainly metal-free things, are really pioneer objects and once they've blazed the trail they’re quickly followed by what we consider to be more conventional stars. And these later generations of stars are likely to be found in even very early galaxies. Indeed, the chemical remnants that have been left behind are by and large not unusual – that is, we have neither seen the first stars directly nor seen direct evidence of the elements they've left behind.
TKF: As you look to the future, what projects does each of you have planned to address these ongoing questions? And as you begin this next phase of work, what is it that motivates your long quest to understand these earliest of times?
ELLIS: Let me address the last question first. The reason I'm working in this area is that it's exciting, and we are actually making progress. If I look at the progress that we have made observationally over the last three years, it's truly amazing.
My personal plan for the next 12 months is two-fold:
First, we have this exquisitely detailed image coming in August (2012) from the Hubble Space Telescope. This will be the deepest image taken by far, with Hubble’s new wide field camera. The new image is expected to reveal objects that are about three times fainter than we can now see, and this will enable us to have a much better understanding of the numbers of galaxies at a redshift of 10 – meaning we’ll be able to see galaxies that formed only 480 million years after the Big Bang. At the moment, there is a lot of controversy about the earliest objects. With the latest Hubble images, we can see perhaps four of these earliest galaxies. With the new image, we expect we’ll be able to see about twenty. So that will be exciting.
The second development I’m looking forward to is the arrival at Keck Observatory in Hawaii of the first multi-object infrared spectrograph. As we've discussed, most of these galaxies between redshifts of seven and ten can only be studied at infrared wavelengths. I think everyone's been hampered, until recently, by the fact that the spectrographs that we use to study the light from these objects can only examine one object at a time. That will change once Keck receives the new spectrograph early in 2012.
So, with several new images from Hubble and the new ability to study several early objects at once, we’ll be able to characterize these objects at unprecedented redshifts and finally see how the dark ages ended. Once we start getting 30 or 40 or 100 objects, I feel confident that over the next year or two we can start to more effectively map how reionization occurred – how the “Swiss cheese” structure we’ve talked about – developed and led to the end of the dark ages.
BECKER: I have two projects coming up in the near future. One is connected to this thermal history that we've been discussing. During reionization the universe is flooded with ultraviolet radiation, which ionizes the hydrogen and heats the gas, and the first thing we try to do is simply to measure the temperature. Well, if you heat gas it expands, and that's also true in deep space. There is a skeleton, a network, of dark matter on which ordinary matter forms galaxies. When the gas is heated, it sort of puffs up and becomes a fluffier version of this skeleton. What we’re going to try to measure next is the degree to which the gas has been puffed up.
This gives you two interesting pieces of information. Number one, it helps you understand both the timing and the patchiness of the reionization process. So again it's an archaeological way of studying the very early universe. Number two, it helps you to understand the way galaxies appear today. One of the consequences of the reionization process that we've been talking about – the heating of the gas – is that it is more difficult for small clumps of warm gas to collect. This is the same phenomenon that Avi was describing with stars. If you heat up the gas in the universe, you will prevent small galaxies from forming. If you look around the Milky Way Galaxy today, you see an absence of some of the small galaxies that we think should be there, based on the dark matter theories that Avi was describing. And examining how the gas heated and puffed up in the very early universe will be one way of connecting reionization, what happens in the very early universe, to the distribution and characteristics of galaxies today.
"The reason I'm working in this area is that it's exciting, and we are actually making progress. If I look at the progress that we have made observationally over the last three years, it's truly amazing." – Richard Ellis
Another project is the pursuit of the most distant quasars that have ever been discovered. With new, deep surveys that cover the entire sky at infrared wavelengths, we are beginning to discover quasars at earlier epochs than have previously been inaccessible. And we are now finding objects that were present when the universe was only about 750 million years old. We’ll be examining both the quasar itself and the galaxy in which it resides, as well as its larger environment – which includes the condition of the gas in the IGM during a time that we think is very close to the reionization epoch, or perhaps within it.
In terms of why I enjoy studying this early time in the history of the universe, I think Richard put it quite well. It's a frontier, and it's a frontier where we are moving ahead. It's a science that really concerns every atom in the universe, and I have to say there's a certain appeal to that.
LOEB: Early on, when I was younger, I was interested mostly in philosophy, in the humanities – and one of the fundamental questions for humans is how everything started. You can find this question discussed in the opening paragraphs of many religious texts, and so the general public is very interested in knowing the answer. It's quite remarkable that modern science has reached a point where we can actually address some of the questions about our origins – how the first light was produced in the universe, and how it led to the formation of everything that we see around us today. So that's what attracts me to this topic, that I can now go full circle and come back to a fundamental question I considered in my youth – addressing it with theoretical tools and actually finding the answer.
In terms of my plans for the next year, I'm finishing up with my former student, Steve Furlanetto – who is by now a professor at UCLA – a textbook called “The First Galaxies,” in which we will summarize the theoretical expectations that we have for what the first population of galaxies looked like. Of course, we don't know if these expectations will be supported by data, but it's good to have them documented so in ten or twenty years it will be interesting to compare the view that we have then relative to the view that we have right now. Any difference would amount to progress, because it implies that we learned a lot. And so it's very important, at this point in time, to summarize everything that we know and expect the early galaxies to have been like, and that's what we are doing in this 500-page textbook that will be published in 2012 by Princeton University Press.
"I enjoy studying this early time in the history of the universe... [because] it's a frontier where we are moving ahead. It's a science that really concerns every atom in the universe, and I have to say there's a certain appeal to that." – George Becker
In terms of research, I'm focusing mostly on the 21-centimeter frontier. I should mention also that I have worked over the last year with a student of mine, Eli Visbal, to come up with an interesting technique to detect spectral lines emitted from galaxies – not only from hydrogen but also from heavier elements. The idea is that by looking across a large area of the sky, you can map the distribution of galaxies without resolving them individually. You are looking for the cumulative emission from all the galaxies, and that includes very small galaxies that otherwise we cannot resolve. The result is a map of the cumulative intensity contributed by all of the galaxies. This is expected to vary from place to place, because the galaxies are clustered and you get fluctuations in intensity of the light emitted by all of the galaxies in those regions. It's similar in spirit to mapping the distribution of hydrogen on large scales using the 21 cm (spectral) line. But now it's different, because it's mapping the emission lines from galaxies without actually resolving individual galaxies.
If we can map the distribution of galaxies and the hydrogen gas between them, in the IGM, we can see the relationship between the places where the galaxies are and the regions that were ionized. In other words, we can understand the full story. Mapping hydrogen gas in the IGM – that is, the holes in that gas – gives us indirect evidence for the earliest galaxies. This new technique will enable us to directly study the galaxies that ionized that hydrogen gas.
There is no observatory at the moment that can detect spectral lines from these earliest galaxies. But several instrumentalists have already contacted me with interest to build related observatories, and I am planning to help them optimize their design.
TKF: So, once you have these two data sets, of the hydrogen gas in the IGM and the spectral lines of the earliest galaxies, they should correlate?
LOEB: There should actually be an anti-correlation, because the regions where the galaxies are will be the first ones to be ionized. There should be a lack of hydrogen around these galaxies, and the places where you find hydrogen you would not find galaxies. And so, the two maps should be anti-correlated.
TKF: Thank you everyone. It would be great to reconnect as we answer some of these big questions about the first stars and galaxies.
LOEB: In ten years let’s talk again, and we’ll see how much things have changed.