Seeing Individual Atoms—And More
by Alan S. Brown
The record for the best electron microscope resolution
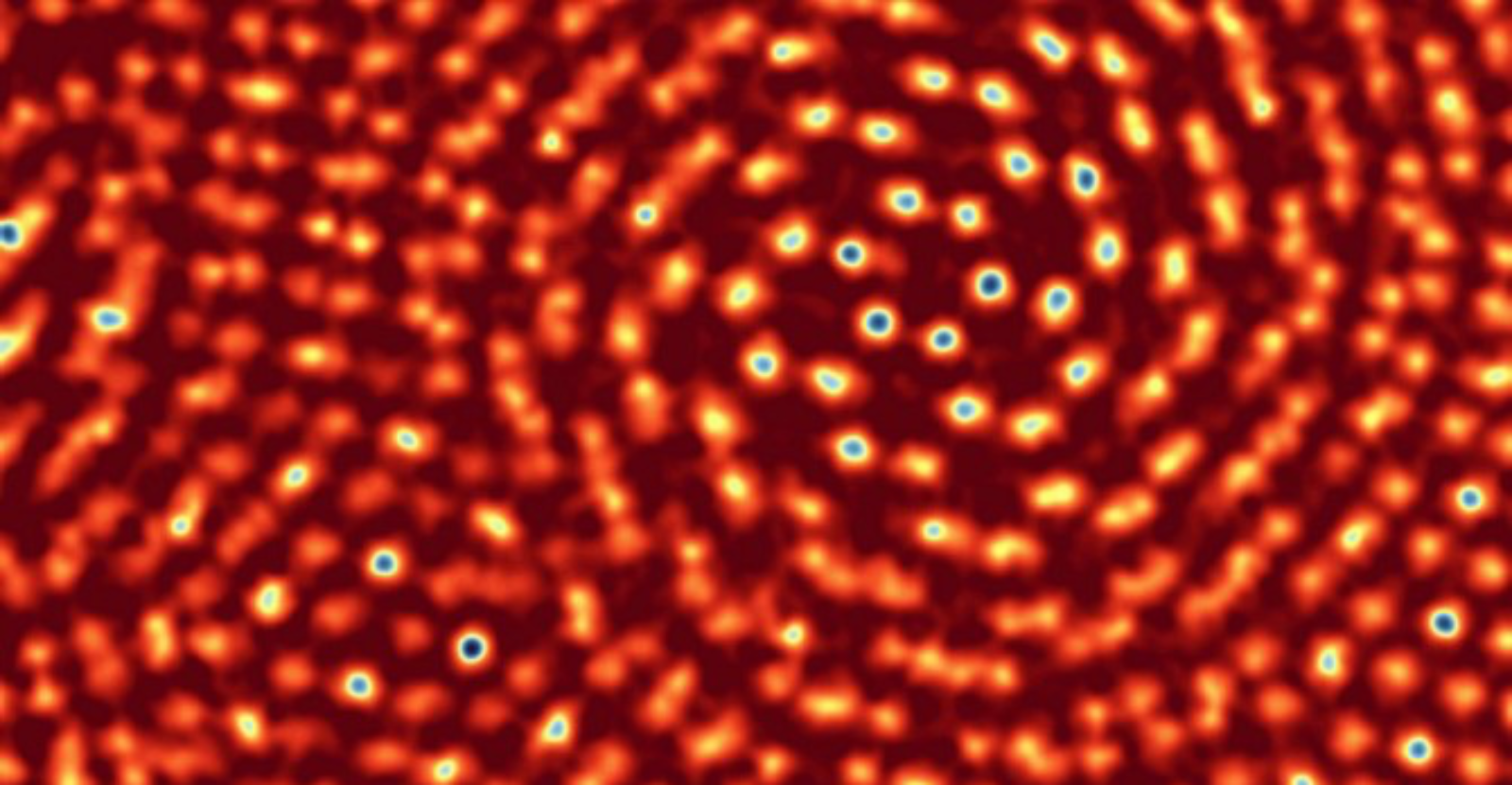
The Author
The Researcher
The Guinness Book of Records is packed with amazing feats, from the fastest 100-meter race run balancing a baseball bat on one finger (14.28 seconds) to the tallest residential building (1,396 feet).
It also lists the record for electron microscope resolution: 0.39 Angstroms (0.039 nanometers). That is three to six times smaller than the bonds between atoms—small enough to image individual atoms.
The record is held by two members of the Kavli Institute at Cornell for Nanoscale Science, co-director Dave Muller and his research collaborator, Sol Gruner.
Their electron microscope pixel-array detector (EMPAD) tripled the resolution of their state-of-the-art electron microscope to reach the new record, but that is just the start of the story.
By analyzing how the electrons scatter as they pass through the sample, the researchers can also capture information about a material’s properties. And, equally impressive, they can study the most fragile of materials—films only one atom thick and proteins—without damaging them with high-intensity e-beams.
E-Beams
Electron microscopes are popular because they see finer details than conventional optical microscopes. The latter use visible light, whose wavelengths were too wide to discern nanoscale details. Think of it as trying to count the threads on a bath towel with your fingers. Electrons, with wavelengths thousands of times smaller, have smaller “fingers” to pick out fine details.
David Muller discovered electron microscopes as an undergraduate at University of Sydney in the late 1980s. He was having trouble characterizing amorphous carbon films. While others were using neutron beams, equipment like that was rare in Australia.
“So, I used the biggest tool I could get my hands on, which was an electron microscope,” Muller said. “It was great. I could measure all the details I wanted. We were getting much better data than the neutron guys.”
He eventually applied to Cornell’s doctorate program. Among its attractions—in addition to snow, which he had never seen before—was access to the school’s new-generation electron microscope. At Cornell and later Bell Labs, Muller explored using electron microscopes to visualize atoms and explore the properties of materials as semiconductor features shrank to just a handful of nanometers.
All the while, electron microscopes were changing. New electromagnetic aberration correctors that fix beam distortions gave resolution a dramatic boost. Unfortunately, each new corrector required exponentially more lenses and controls. This proved unwieldly.
As resolution improved, researchers targeted ever finer samples. This led to a second problem: many objects were too fragile to withstand the powerful e-beams.
Muller and Grunner found a way to solve both problems at once.
Staring into Headlights
After Bell Labs, Muller joined Cornell. There, he met Gruner, who was working on direct detectors for X-ray synchrotrons.
Direct detectors are arrays of sensors, called pixels, that register the charge of an electron (or presence of an X-ray) when it strikes them. Unlike the indirect detectors used on electron microscopes, which translate electrons into light for measurement using a scintillator, the direct detectors are sensitive enough to easily detect even single electrons.
Muller thought Gruner’s sensitive detector might improve his electron beam microscope, so he and another student tried it out.
“The electron microscope totally saturated the detector,” Muller said. “What took two hours on the synchrotron took under one second. It couldn’t handle the fluxes.”
It took a decade to develop a solution.
“Instead of trying to count every individual electron—they’re coming too fast to do that—try to imagine that each pixel on the detector is a bucket,” Muller said. “Then I come in with a mug and scoop out 20 electrons at a time and count what is left in the bucket. As long as I’m scooping electrons faster than they are coming in, the bucket doesn’t overflow. It never saturates.”
Scooping electrons happens so fast, some pixels are empty while others were always filled. The variation in signals between the strongest (always full) and weakest (always empty) made the sensor incredibly sensitive. A camera with a similar dynamic range could show the license plate numbers of a car at shining its lights on you at night.
Direct detection also worked with gentler, lower powered electrons. In fact, an EMPAD scan of a one-atom-thick film of molybdenum disulfide could pinpoint missing sulfur atoms, something very hard to do with other electron microscopes.
By capturing scattered electrons and working backwards, he could also map the magnetic and electrical fields of objects as well as nanoscale strains buried deep within material structures.
Cornell licensed EMPAD to Thermo Fisher Scientific, a large maker of scientific instruments. Muller sees applications everywhere, from investigating semiconductor nanostructures and exploring nanoscale magnetic fields to investigating the structure of proteins.
EMPAD is, indeed, quite a breakthrough. In fact, it’s one for the record book.